A Physicist’s Approach to Biology Brings Ecological Insights
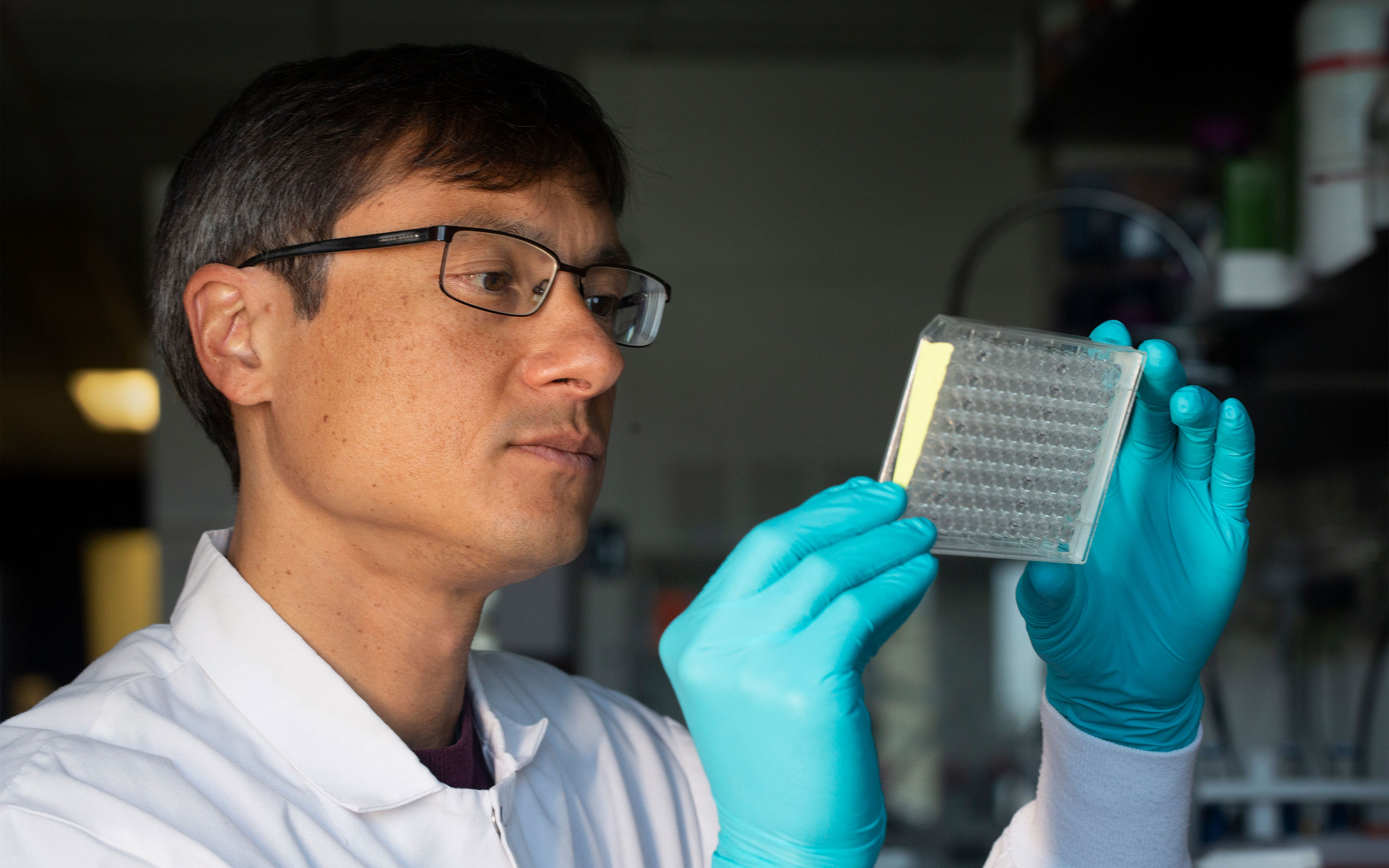
Jeff Gore of the Massachusetts Institute of Technology is a physicist by training, but he brings the rigorous experimental practices of that field to his research into principles guiding the dynamics of micro-ecologies.
Katherine Taylor for Quanta Magazine
Introduction
Last year, Jeff Gore strode down a short hallway from his office to his laboratory in one of the university buildings on Technology Square in Cambridge, Massachusetts. Machines hummed, and a faint chlorine smell provided assurance that things were being kept properly sterile. Lab counters were strewn with pipettes and roughly smartphone-size plastic plates that held 96 semi-spherical wells, each about a half-centimeter in diameter, in 8-by-12 grids. Gore picked up one plate. “This is like our world,” he said.
Members of Gore’s laboratory at the Massachusetts Institute of Technology spend their days creating, poking and prodding isolated islands of single-celled bacteria or yeast living in each of those wells. Species are pitted against each other or subjected to heat, cold or food shortages. All the while, devices called cytometers read out the results of those thousands of experiments, well by well, sometimes even cell by cell. For Gore, being able to precisely quantify the results is the key. “You can really count cells,” he said.
The surroundings and research subjects befit a biologist. But by both training and self-identification, Gore is a physicist. And with the audacity that makes physicists both valued and at times resented when they trespass into other fields, this fast-talking, perpetually upbeat physicist has for the last decade conducted what he hopes will be a beneficent invasion of ecology.
Gore has built a research career on manipulating stripped-down ecosystems of microbes in search of the kinds of fundamental, universal principles that physicists have made their calling card when it comes to the inanimate world, and that ecologists have long sought for the living one. What sets his approach apart, colleagues say, is the pursuit of reproducible experimentation and statistical analysis that ecologists might traditionally have regarded as potentially unnatural and misleading, if not outright unattainable.
The approach has succeeded perhaps more than it had any right to. Gore has discovered rules that allow him to make predictions about simple micro-ecological systems, including the gut microorganisms of laboratory worms and bacterial communities in samples of soil. Even biologists and ecologists who might be inclined to mistrust an intruding physicist praise Gore’s respectful attitude toward their field and say that he’s making real headway on problems that have long confounded them and their colleagues.
Gore “can ask really profound questions where people have scratched their heads for decades,” said Stefano Allesina, a theoretical ecologist at the University of Chicago. “He can distill theory into a beautiful experiment where you can get a clean signal, clean data.”
Now Gore is pushing into new territory. Having mastered the manipulation of microbes in the lab, he hopes to discover the rules that govern real-world ecosystems that people actually care about. Understanding how species assemble and persist in communities could help doctors treat intestinal disease, enable agronomists to engineer healthier soil, and help conservationists protect or restore ecosystems.
But colleagues on both sides of the physics-biology divide, while supportive of the effort, wonder how Gore’s radically austere experimental strategy will fare when confronted with the full complexity of the living world.
Explaining the Tangled Bank
When contemplating the deepest questions in their field, physicists may ask: Why is there something instead of nothing? Ecologists often ask: Why are there many species instead of just a few?
The legendary ecologist G. Evelyn Hutchinson called it the paradox of the plankton, but he could just as well have called it the paradox of the prairie, the paradox of the rainforest or the paradox of the gut microbiome. Practically everywhere you look, nature chooses complexity over simplicity, medleys over monocultures. The world is full of species, but it’s not clear why: In any given environment, why doesn’t one species (or at most a few) gain an advantage and outcompete all the rest? The problem has vexed scientists for more than 150 years, ever since Darwin famously mulled the “tangled bank” in On the Origin of Species.
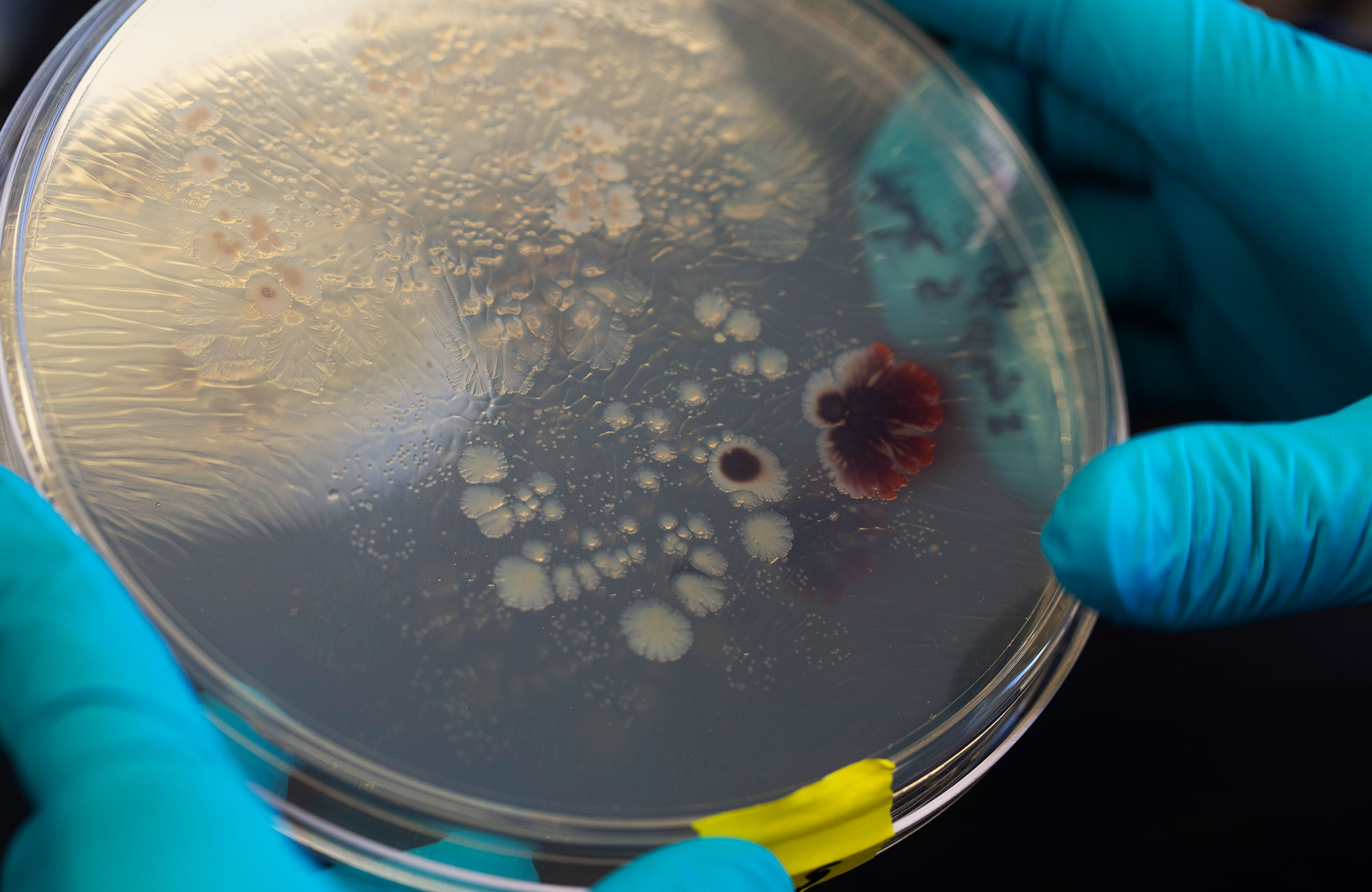
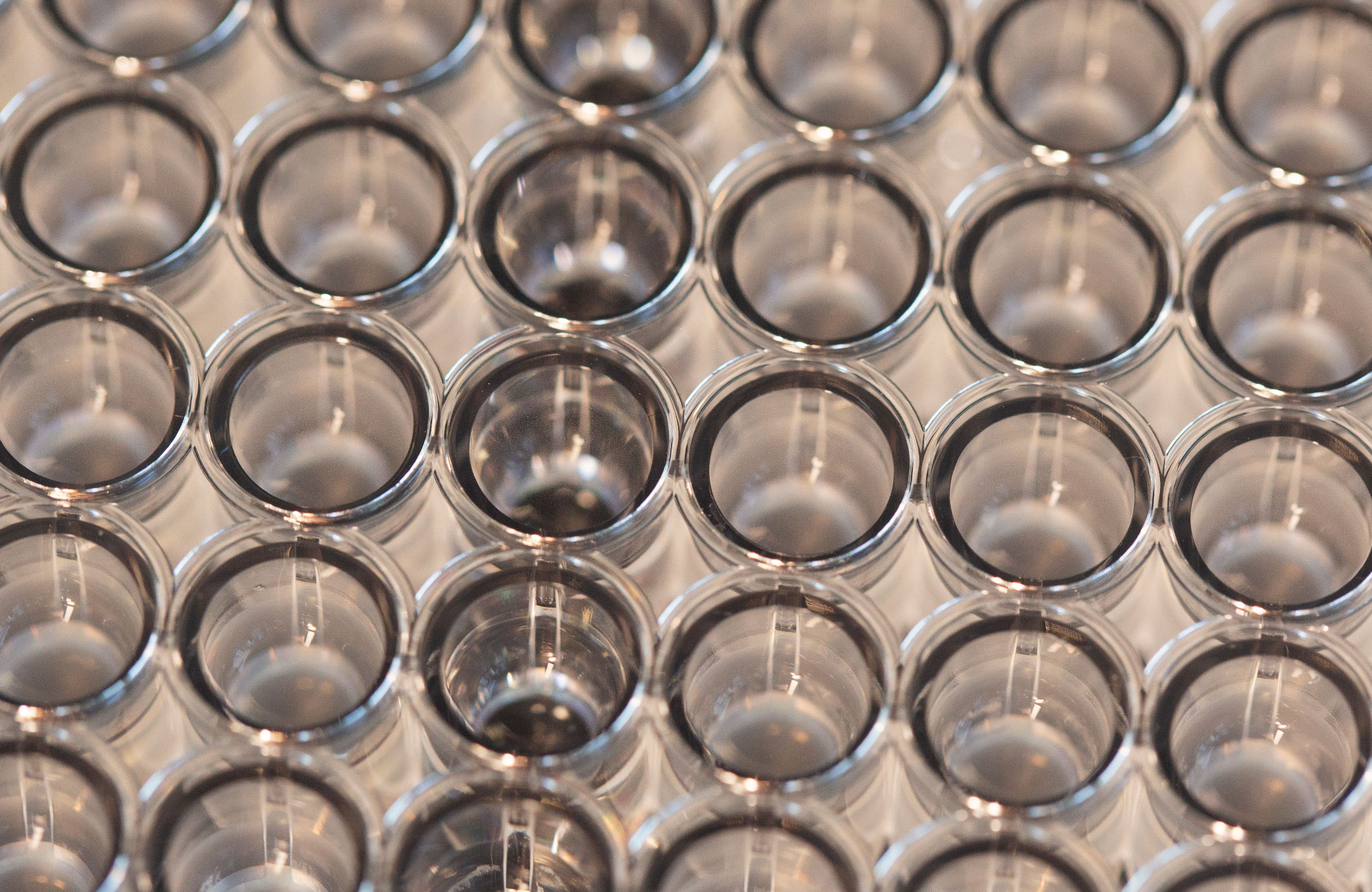
Researchers can grow communities of microbes on culture dishes to observe the stability and competitiveness of the species (left). Gore and his colleagues rigorously and systematically test the results of various combinations of microbes in 96-well culture plates (right) to determine rules for the outcomes of different pairings.
Katherine Taylor for Quanta Magazine
In the 1920s, the mathematical biologists Alfred Lotka and Vito Volterra independently wrote pairs of coupled equations to explain how a predator species and its prey could cause each other’s numbers to oscillate stably rather than explode or collapse, providing theoretical support for coexistence in a simple ecosystem. But more complex ecosystems defied such clean mathematical treatments.
As ecology matured into a distinct discipline, work by Hutchinson and others spawned a more qualitative idea called niche theory. Its general concept is that species thrive by being the best at capitalizing on a particular set of environmental conditions — some trees prefer cool, wet sites while others do better in hot, dry ones; a third set germinates only after a fire, and so on. Nature’s heterogeneity therefore carves out spaces for a profusion of organisms.
Around 20 years ago, niche theory’s dominance was challenged by neutral theory, which posited that differences between species don’t generally matter ecologically because speciation, extinction and the death of individuals are more random than is usually supposed, and that randomness keeps any species from dominating its rivals. The debate rages to this day — though few ecologists fully reject the niche concept.
The frustration for theoretical ecologists is that, with thousands of disparate ecosystems around the globe, ecological theories can be hard to universally falsify. Allesina jokes that physics textbooks stay at about the same length over time because experiments and observations falsify old theories at the same time that they catalyze new ones. But ecology texts grow ever longer, because older theories stick around even as new ones pile up. It’s unclear whether unifying principles that underpin ecology — the equivalent of thermodynamics and statistical mechanics in physics — are unknown because no one has yet uncovered them or because such principles simply don’t exist.
Testing the Big, Beautiful Theories
Gore, both physically and intellectually restless, entered the ecological fray after dipping his toes in several other scientific ponds. As an undergraduate at MIT in the late 1990s, he helped to develop an experimental apparatus for studying Bose-Einstein condensates with Wolfgang Ketterle, who would go on to win a Nobel Prize in 2001 for being among the first to produce that long-theorized fifth state of matter. In graduate school at the University of California, Berkeley, Gore migrated to biophysics, probing the complicated mechanics of the DNA molecule. He returned to MIT as a postdoc, and this time he pushed beyond the boundaries of conventional biophysics, using yeast to experimentally probe the evolution of cooperative behavior.
Over time, Gore was seduced by what he calls ecology’s “big, beautiful theories” for how living beings can exist in complex communities. But he also noticed that “theory and measurements are not always enriching each other as much as they could.” He saw an opening for clear, unambiguous and replicable measurements that could rigorously test hypotheses and perhaps chip away at the theoretical pileup that Allesina laments. To Gore, that meant exerting a level of control over nature that many biologists might find artificial in the extreme. He began putting microbes in tightly controlled settings and using cutting-edge instruments to precisely track the fates of individuals. This, he reasoned, would allow him to tune out some of the noisiness and chaos of nature so he could replicate experiments many times and generate the kinds of statistics that convince physicists they’ve nailed down a phenomenon.
“Jeff’s take on this was: Let’s take the most simple thing, and let’s see how well we can measure this most simple thing,” said Kirill Korolev, a physicist who did research in Gore’s lab and now runs his own at Boston University. “You lose complexity, but there are also benefits.”
Gore began with the simplest possible ecosystem: just one species. Even that, it turned out, could be interesting. Gore and his lab members, including Korolev, designed an experiment in which they diluted colonies of brewer’s yeast growing in sugar solutions. Brewer’s yeast cells convert inedible sugars into edible ones, so individuals benefit from having many close neighbors, while dilution starves yeast populations. The researchers identified signals in their data allowing them to predict whether the yeast populations would thrive or collapse. The study, published in 2012 in Science and the most cited paper from Gore’s lab to date, featured a figure known as a bifurcation diagram, which maps the precise conditions under which the ecosystem stays stable or passes a catastrophic tipping point.
Such a diagram is “something that is very dear to most mathematical ecologists,” Allesina said. The late Robert May, a pioneering physicist turned ecologist, helped launch the field of chaos theory when he discovered that an equation used to model the population growth of insects produced a bifurcation diagram. Gore’s team took the idea from the computer and the blackboard into the lab.
“That paper was super striking,” Allesina said. “The data show that it works exactly as theory would predict. This was the thing that made me very, very aware of [Gore’s] work.”
Between Two and Infinity
There’s a joke that physicists can describe systems of one, two or infinite individual elements, but nothing in between. So having proved his mettle generating insights from one species, Gore moved on to two. In work led by then-postdoc Jonathan Friedman, members of Gore’s lab isolated eight bacterial strains from soil and pitted them against each other in various combinations in their 96-well plates. From the outcomes, the researchers built up a set of simple rules: A outcompetes B, B outcompetes C, C and D can coexist, and so on.
The scientists then mixed the species in trios. Gore’s team found that the outcomes of the one-on-one battles allowed them to predict with 90% accuracy whether a given species would survive in the more complex mixtures. In other words, if A beat B, and B beat C, A would almost certainly beat B and C.
Still, eight microbes hardly prove a universal point. So the researchers took another step toward the real world: Graduate student Logan Higgins went outside to the lawn of MIT’s Technology Square and scooped up some soil teeming with microbes. The team isolated strains and determined the pairwise rules governing the microbes’ abilities to outcompete or coexist with one another. The researchers then looked at almost a thousand bacterial trios in their plastic wells.
These experiments were motivated in part by suggestions dating to the 1970s that mixtures of many species might stabilize each other through competitive balance. One example of such a “higher-order” stabilizing interaction resembles a rock-paper-scissors game, in which each of three coexisting species suppresses one competitor but is suppressed by the other. Theorists have proposed that such arrangements could be an elegant way to generate diverse ecosystems by preventing any one species from outcompeting the rest. Bacterial trios stabilized by rock-paper-scissors relations have even been engineered in the lab.
But a dearth of empirical data has made it hard to tell how important this arrangement is in the wild. “I love rock-paper-scissors,” Allesina said. “That doesn’t make it very common in nature.”
Among the soil strains, Gore’s team found just one rock-paper-scissors relationship. As in the earlier experiment, the outcomes of the one-on-one battles seemed to govern the overall ecosystem; in only one case did bacterium C beat A to complete the triangle. When the researchers threw 20 strains into wells all together, just three emerged as dominant, as predicted by the pairwise rules — a far cry from the original diverse, stable soil community. With rock-paper-scissors almost entirely ruled out, Gore’s team concluded that tiny shifts in environmental conditions within the soil’s physical matrix probably stabilized the diverse community in its natural setting, because relationships of three or more microbes did not seem to be doing the job in the lab.
Lucy Reading-Ikkanda/Samuel Velasco/Quanta Magazine
The studies hinted that Gore’s methods could have something to say about the no-physicist’s-land between two and infinity that real-world ecosystems occupy. “I don’t think that what we find necessarily implies this is how any ecosystem works,” said Friedman, now a senior lecturer at the Hebrew University of Jerusalem in Rehovot, Israel. “But it is the best effort at figuring out how one ecosystem works.”
“We’re really building up … similar to the way that physicists understood quantum mechanics and atoms,” Gore said. “We first tried to understand hydrogen. It’s not that physicists were obsessed with hydrogen per se, but if you don’t understand hydrogen, it’s a little dangerous to try to go too far. … That’s how I view our approach. We started with hydrogen, and we’re kind of at helium and methane right now.”
Building Up
Microbes are vital to nature. Soil bacteria and fungi sustain the plants we eat and the forests that provide climate stabilization and most of Earth’s biodiversity. In our guts, bacteria help us digest food and are thought to contribute to immunity and possibly even cognition. But microbial communities can also cause devastation. Perhaps the best-known example occurs when the bacterium Clostridium difficile invades the gut, causing painful and sometimes deadly colitis. Such invasions are difficult to predict and difficult to reverse. Today, they are increasingly treated via fecal transplant — a brute-force attempt to replace an unhealthy microbiome with a healthy one.
“We don’t understand anything, so we transplant the whole community and cross our fingers,” Allesina said. “Do you really think you need the whole 5,000 strains? … Probably not. Probably smaller communities that have only a handful of things would have the same outcome.”
Buoyed by his string of promising results, Gore has lately set his sights on the gut microbiome. In a study published in February, his team found that when a two-microbe ecosystem was briefly invaded by a third species, the dominant strain in the ecosystem often switched. A similar dynamic, the authors suggested, could be at play in C. difficile infections.
But in trying to distill universal species assembly rules from the morass of the human gut, Gore faces a daunting climb in complexity. In our guts, more than a trillion bacteria comprising hundreds of species compete and cohabitate at any one time. A pathway toward progress opened when a new postdoc, Nic Vega, brought a worm to the Gore lab: Caenorhabditis elegans, a model organism studied by thousands of biologists. The worm, like us, has a gut and a microbiome, but that microbiome has a mere 100,000 or so members.
Adapting the approach from the soil experiments, Vega fed worms pairs of red- and green-labeled bacteria, let the bacteria duke it out inside the gut, and then ground up the worms to determine how much of each type survived. They determined that random fluctuations in the rates at which the two bacterial species colonized the worms led to sizable differences in the worms’ fully assembled microbiomes. The idea that such differences could be explained, Vega said, was “a bit alien” to microbiome researchers.
In a follow-on study, Vega and graduate student Anthony Ortiz Lopez studied the outcomes of pairwise competitions among 11 bacterial species that live in worm guts, and then fed worms mixtures of up to three of these species. As in the soil, pairwise rules largely predicted the final outcome in most cases. The researchers posted a preliminary paper on the biorxiv.org preprint server and have submitted it to a journal for peer review.
The experiment demonstrated that although external factors matter, “pairwise outcomes are powerful objects” for predicting the evolution of microbial communities in a range of environments, Gore said.
Similarly, to Vega, now a professor at Emory University in Atlanta, the result represents a powerful demonstration that Gore’s methods translate to real-world organisms. “Certainly in a human gut ecosystem, it’s not physically possible to do all pairwise interactions of the microbes that exist,” Vega said. “What we’re trying to figure out now is … what information do you actually need to predict the behavior, and what’s the best way of getting it?” What Vega has seen so far makes them optimistic. “I do have hope that we’ll be able to understand the properties of the mammalian microbiome, and intentionally re-engineer the mammalian microbiome.”
Not everyone is convinced of this. Beyond the difficulties created by their sheer numbers and diversity, bacteria constantly secrete compounds that change the environment both for them and for everything around them. Capturing such nuances will require advances beyond what Gore or anyone else has achieved.
“I think it’s going to be very difficult to completely replicate human microbiomes in the lab,” said Tami Lieberman, an MIT biologist who studies microbiome-based therapies. But, she emphasizes, that doesn’t mean it’s not worth the effort. Indeed, as a start in that direction, Lieberman and Gore are discussing a collaborative experiment to test how well pairwise rules predict microbial interactions in the human gut.
For Pankaj Mehta, a theoretical physicist at Boston University, just across the Charles River from MIT, Gore’s approach has exceeded expectations. Early on, Mehta thought Gore was ignoring important details about microbial environments, but Mehta concedes that these details have proved less important than he believed. “What’s interesting and impressive about the work is that they show that many, many different phenomena — pH changes, competition for nutrients — can all be captured using these simple phenomenological models, at least in systems where you have a few species,” Mehta said. “This is a true and tried reductionism of the sort that’s served well in physics.”
But Mehta, too, remains skeptical about how far Gore can go. He provides an analogy: When physicists try to describe 1023 air molecules that might whiz around a room at any given moment, they do not write an equation for each molecule. Rather, they seek parameters that describe the particles’ average behavior — hence the infinity in the “one, two and infinity” joke. For a gas, it turns out such a parameter exists: temperature. Look at a thermometer, and you’ll know a lot of what’s worth knowing about any sufficiently large number of molecules around you.
Mehta believes this “top-down” approach can also work for microbes. In studies his group and others have carried out, high-level parameters such as the availability of nutrients and the degree to which bacterial species depend on each other’s secretions captured important information about how microbial communities function, without requiring detailed knowledge of those communities’ members or interactions.
Gore, Mehta says, effectively tries to account for every microbe, in what scientists call a “bottom-up” approach. While that has worked well so far, “I do believe it will be undoubtedly much harder to induct a way to very complicated systems,” Mehta said. “I certainly think it will become less and less predictive.”
Allesina, in his own work, also favors the top-down approach. In a 2018 modeling study, he threw 100 simulated microbes together and found that stable ecosystems formed regardless of the specifics of inter-microbial interactions. But Allesina believes both strategies are needed. “I like the top down … it’s more fun,” Allesina said. “But I think the answer will be in the middle.”
The Princeton theoretical ecologist Simon Levin notes another challenge Gore must contend with: Microbes, unlike chemical elements or subatomic particles, are constantly evolving, so your study system changes while you’re experimenting on it. “That’s always going to be a limitation of any sort of predictive theory” in biology, said Levin. “When something can come in from left field, it’s very difficult to build a theory of what it might be.”
The next few years may show whether Gore can achieve the lofty goals he has set for himself. True to his restless, improvement-seeking nature, he has lately become excited about a collaboration with Friedman and Paul Blainey of the Broad Institute that repurposes a nanofluidics chip Blainey built for high-throughput drug screening. The researchers recently used the chip to study more than 180,000 interactions among 20 soil bacteria in 40 different environments and found that interactions in which one microbe benefits from the presence of another are much more common than expected — a result that could be another blow to conventional wisdom about how bacteria behave in communities.
Levin says Gore has increased his odds of success by doing one important thing right. “He doesn’t come in like a lot of physicists do and say, ‘I’m a physicist and I’m here to help you,’” Levin said. “Jeff has converted himself into a biologist. He shows a respect for the ideas of the field.”
Allesina agreed. “We’re lucky that he decided to study ecology.”
This article was updated after publication with the video of Jeff Gore.
Correction: October 14, 2020
The video accompanying this article originally neglected to credit some footage to “Journey to the Microcosmos.”