Cellular Life, Death and Everything in Between
Introduction
“When cells are no longer needed, they die with what can only be called great dignity,” Bill Bryson wrote in A Short History of Nearly Everything. The received wisdom has long been that this march toward oblivion, once sufficiently advanced, cannot be reversed. But as science charts the contours of cellular function in ever-greater detail, a more fluid conception of cellular life and death has begun to gain the upper hand.
Perhaps the most dramatic proof of this emerged last April, when a team at the Yale School of Medicine drew global attention for briefly restoring cellular activity in dead brains. The neuroscientists Nenad Sestan, Zvonimir Vrselja and their colleagues developed a system called BrainEx that can perfuse a brain with a hemoglobin-based solution to nourish cells while promoting their recovery from oxygen deprivation, a condition that is usually lethal for neurons after 10 minutes or so. They tested it on brains extracted from slaughtered pigs, deprived of blood and kept at room temperature for as long as four hours — making them quite thoroughly dead by any conventional standard.
Yet after being perfused with the experimental solution for six hours, many of those deteriorating and seemingly lifeless brain cells regained — at least temporarily — some of their normal structure and metabolic activity. Slices of neural tissue were even able to conduct electrical signals (for ethical reasons, the researchers had generally suppressed that capacity in the animals’ whole brains). BrainEx or something like it might someday be used to restore brains threatened by strokes, oxygen deprivation or other conditions, although years of further testing will be needed before that’s possible.
The Yale team’s achievement headlined a spate of recent work that probes the liminal space between cellular life and death. Although life and death are commonly treated as alternatives, it’s not that simple. Physicians, medical ethicists and lawmakers have struggled to define the moment of death for humans: Is it when breathing ceases? When the heart stops beating? When brain activity is undetectable? Different answers arise because death is a process, and not necessarily an irreversible one.
The barrier between life and death is porous even at the level of cells, the fundamental units of life. Researchers are learning that cells once thought terminal or dead can resurrect themselves, or be partially resurrected, under the right conditions. By studying the processes that allow cells in limbo to inch back toward life, scientists ultimately hope to restore diseased cells to health, limit overactive cell division, resuscitate expired organs and even pave the way for long-extinct creatures to roam again.
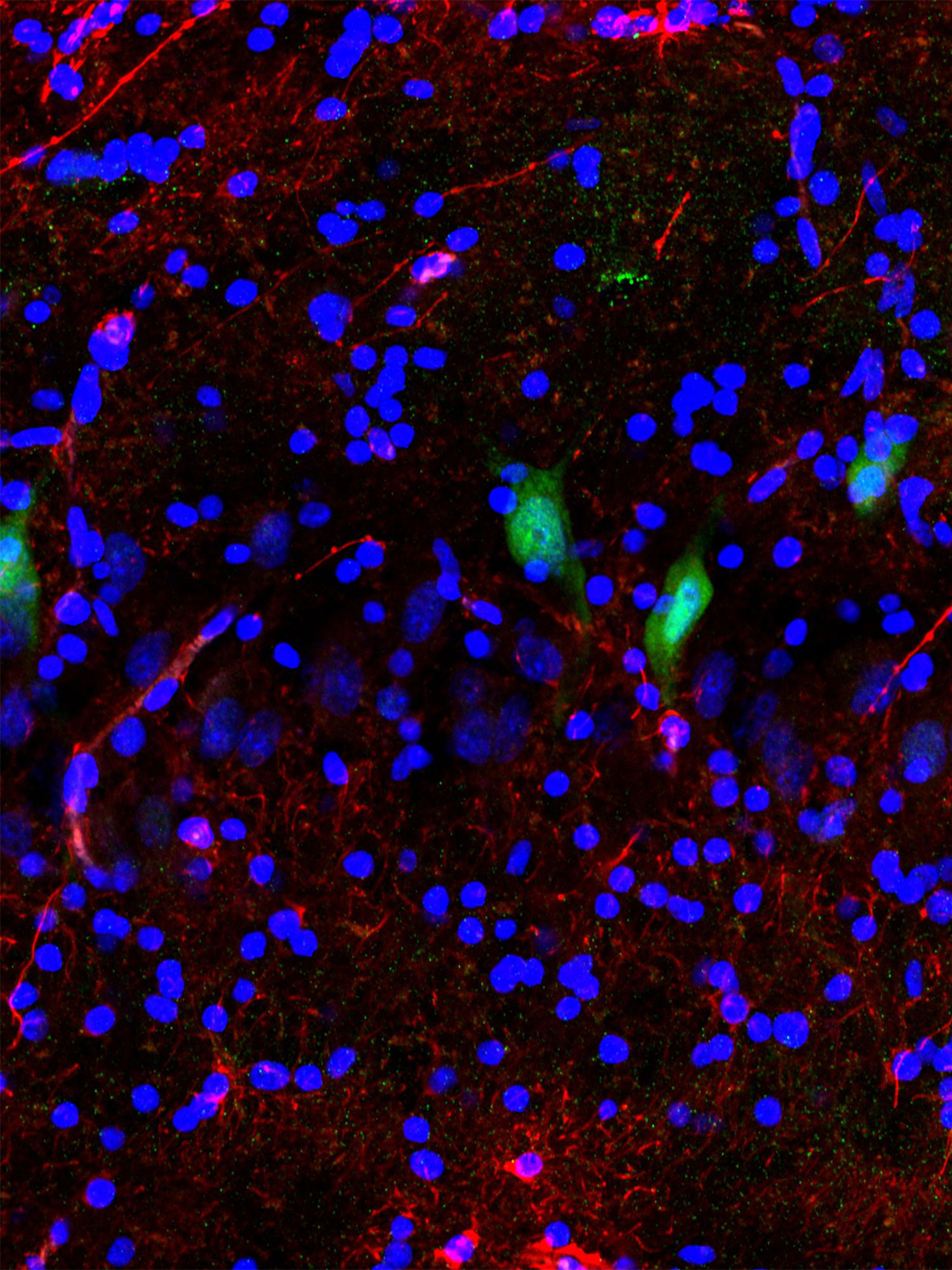
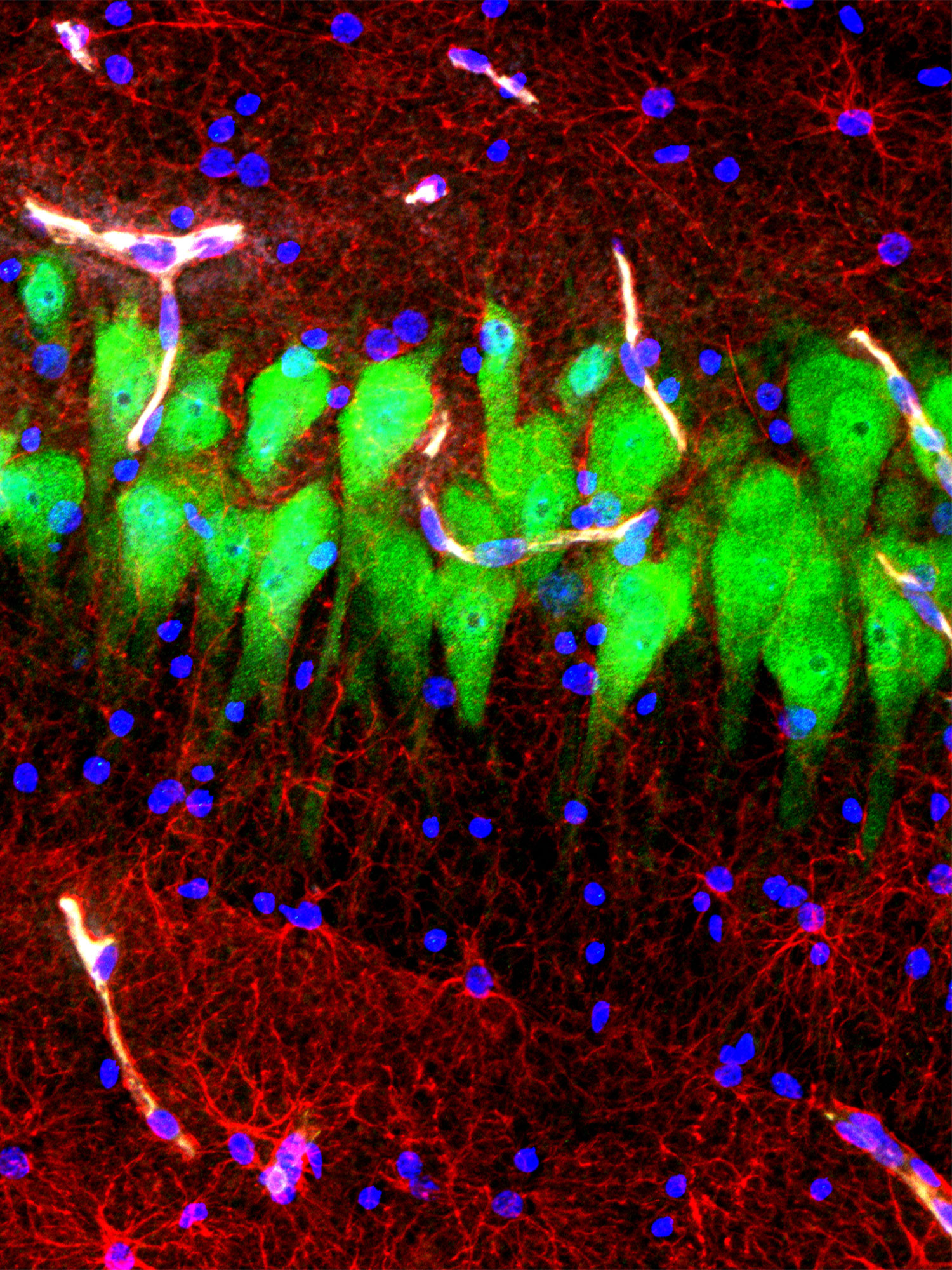
Ten hours after death, cells have largely disintegrated in a micrograph of untreated brain tissue (top) but have been salvaged in tissue treated with the BrainEx system. In these immunofluorescence images, neurons appear green, astrocytes are red and cell nuclei are blue.
Ten hours after death, cells have largely disintegrated in a micrograph of untreated brain tissue (top) but have been salvaged in tissue treated with the BrainEx system. In these immunofluorescent images, neurons appear green, astrocytes are red and cell nuclei are blue.
Ten hours after death, cells have largely disintegrated in a micrograph of untreated brain tissue (left) but have been salvaged in tissue treated with the BrainEx system (right). In these immunofluorescence images, neurons appear green, astrocytes are red and cell nuclei are blue.
Stefano G. Daniele & Zvonimir Vrselja; Sestan Laboratory; Yale School of Medicine
Back From the Brink
Intuitively, we tend to think of resurrection as a spectacular, showy event: a phoenix rising from the ashes. But on a cellular level, resurrection is less a triumphant transformation than the crescendo of a finely tuned orchestra.
While it’s easy to equate death with chaos, cell death is far from a random process — cells that are about to expire tend to shut down in a predictable way. Their internal generators slow, their organelles may fragment, and their DNA breaks down.
To mount a resurrection bid, cells must reverse these processes and attempt to repair the damage through a complex, tightly choreographed operation. They can often jump-start this intricate recovery on their own — a discovery that emerged several years ago as scientists questioned old assumptions about the finality of cell death.
Among the most persistent initial questioners were the siblings Ho Lam (Hogan) Tang and Ho Man (Holly) Tang, biologists who were then at the Chinese University of Hong Kong. The Tangs had long heard that once cells enter apoptosis, a classic death sequence many cells go through, they cannot be brought back to life because of caspases — the so-called executioner enzymes that chop cellular proteins into fragments. Caspases were considered the final death knell for almost any cell: Once they appeared, there was supposedly no way apoptosis could be reversed. But Hogan and Holly Tang weren’t so sure. “We asked, ‘Is this really true?’” Hogan Tang said.
To find out, in 2008 the Tangs designed a simple experiment. They immersed human tumor cells in ethanol to kick-start apoptosis. But instead of tossing out the presumably dead cells afterward, they washed the cells and put them in a fresh culture medium.
The results were shocking. By the next morning, some of the cells had regained their normal, healthy appearance. “It’s not something we expected,” said Hogan Tang, who is now at the Johns Hopkins School of Medicine. In place of the shrunken, shriveled cells they had left, they found flush cells with intact membranes. Key organelles such as mitochondria were functioning normally again. All signs pointed to a dramatic reversal overnight — a cellular greatest comeback.
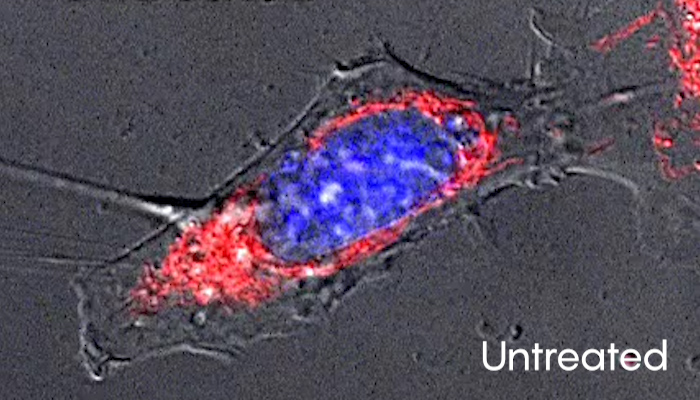
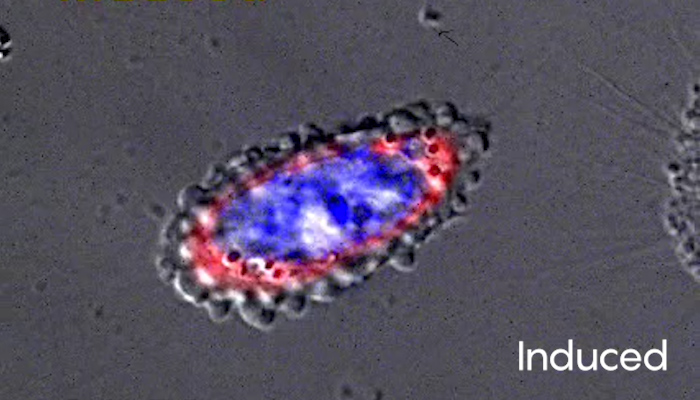
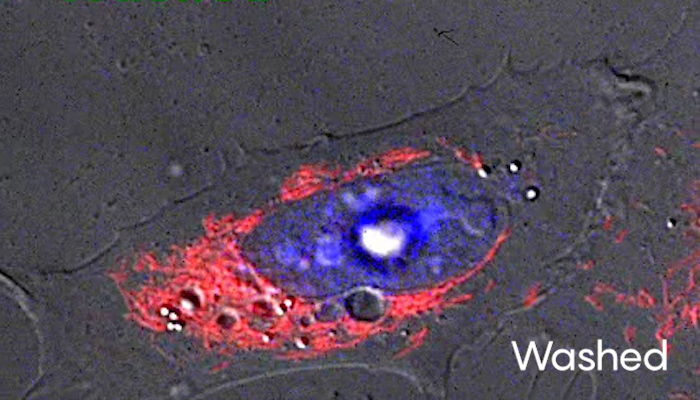
Images capture a mouse liver cell’s induced death and recovery through anastasis. Before treatment, the cell looks healthy. After 2.5 hours in medium containing ethanol, it has lost most of its structure. Washing and incubating the cell in fresh medium for an hour then returns it to a more normal appearance. Click on the second and third images to watch time-lapse video of those changes.
Courtesy Ho Lam Tang
When the two scientists identified the enzymes active during this process, they confirmed that cells could recover even after caspases had begun to chop up their innards. In 2012, Hogan and Holly Tang dubbed this cellular recovery process “anastasis,” from the Greek word for “rising to life” or resurrection.
The cell biologist Denise Montell — who worked with the Tangs at Johns Hopkins and was a co-author on the 2012 paper — had also begun questioning the existence of a cellular point of no return. To investigate, Montell, now of the University of California, Santa Barbara, submerged some human cancer cells in alcohol to initiate cell death. The alcohol had the expected effect within a few hours: The cells began to shrink, their membranes bulging like water balloons with weak spots.
After Montell’s team washed the cells to remove the alcohol, they tracked the cells’ anastasis by the hour. Initially, she thought the cells had completely recovered after just two hours, but after 12 hours she realized that the cells looked much different than they had 10 hours before. Sequencing genes that were active at various time points revealed that “basically, there’s two phases to the recovery,” Montell said. “There’s a later phase that involves different processes.”
In the first phase of anastasis, cells whose growth has been stopped during apoptosis begin to grow again and resume a normal cell cycle. In the later stage, after about half a day, the cells begin to elongate and move around more than before as they divide and reproduce — a response that, in live animals, could help damaged tissues fill in and regrow. Montell also noticed that genes promoting growth and recovery were active during apoptosis to some extent, suggesting that the seeds of potential anastasis are sown even as apoptosis begins. “Cells poise to recover even as they are dying,” she said.
In 2015, through work started in Montell’s lab and then continued in that of J. Marie Hardwick at Johns Hopkins, the Tangs unveiled a way to track anastasis in living organisms by tagging animals’ genes with a fluorescent protein that glows only after the caspase enzyme is activated. That allows them to see which cells progress partway through apoptosis, then reverse gear and return to normal function. The researchers have used various techniques to demonstrate that anastasis occurs in organisms ranging from fruit flies to rodents. This is “quite shocking,” Montell said. “These events are not rare even during normal development.”
That suggests anastasis may persist because of the evolutionary advantage it offers. “Sometimes cells are exposed to a severe but temporary stress, and it might not be advantageous for every cell in your body to commit suicide,” Montell said. “It evolved as a way to limit permanent tissue damage.”
If some cells die in the face of nutrient shortages, for instance, more nutrients will be available for the surviving cells to consume — provided they can reverse the death sequence. When Hogan and Holly Tang gave fruit flies a protein-rich feast after days of deprivation, fly egg cells that had started apoptosis reversed course and repaired themselves. Anastasis, then, is a counterweight to the cellular pruning bodies perform under stress, an energetic yang to the yin of apoptosis.
A Shrewd Awakening
While anastasis is a relatively recent discovery, a subtler form of resurrection has intrigued biologists for decades: the process cells use to dredge themselves out of deep dormancy. In a wide range of species, certain cells can slow down their activity dramatically, like lights that dim without quite going dark. As they linger in this quiescent state, they wait for the right moment to come back to life — whenever conditions are ideal for them to flourish.
One of the best-studied dormancy exemplars is the wood frog, Rana sylvatica, which freezes solid when the temperature plummets in winter. Before the first freeze, the frog finds a nest of leaf litter in which to keep itself insulated. As ice crystals spread throughout its body, its liver churns out glycogen, a natural antifreeze that prevents cells from bursting. That adaptation fortifies the cells against freeze damage so that most of them can resume normal activity later.
Researchers like Kenneth Storey are working to understand how these cryo-preserved frog cells manage to return to full function. Storey, a molecular biologist at Ottawa’s Carleton University, has studied what he calls “frogsicles” for many years, and gene sequencing has helped him identify certain underpinnings of their remarkable recovery.
When the frogs enter their prolonged stasis, as Storey found in 2016, their cells secrete a substance called transforming growth factor-beta (TGF-beta), which spurs the production of proteins that bring cellular processes to a halt. At the same time, these proteins activate specific genes that assist in blood clotting. That helps ensure that any minor bleeding during the freezing process will be controlled during dormancy, allowing the frog’s tissues to recover more quickly when the long winter ends. Antimicrobial proteins also build up in frozen frog cells, keeping the cells healthy enough to mount a strong comeback in the spring.
While few animals use the same cold-survival methods as wood frogs, mammalian cells do employ similar self-protective strategies when they go dormant. The cell biologist Laila Ritsma studies dormant cells in live mice at the Leiden University Medical Center in the Netherlands using an imaging technique called intravital microscopy. She stains her cells with fluorescent dye, which gets progressively more diluted once the dyed cells start to multiply. “If a cell proliferates a lot, you will end up with very little dye,” Ritsma said.
In dormant cells, however, the dye stays intensely concentrated, allowing Ritsma to identify these cells by sight. Using fluorescent genetic labeling, she tracks the fate of individual dormant cells over time. During dormancy, “the metabolism of cells starts to be low — they shut down all the pathways that are required to proliferate,” Ritsma said. “But it’s not true that they don’t do anything.”
Ritsma’s investigations suggest that, like dormant frog cells, dormant mammalian cells cleverly optimize their chances of successful revival, hunkering down and then roaring back when conditions are best. She reported this year that, based on protein expression studies, mammalian cells use the TGF-beta protein to control their dormancy status just as wood frog cells do. “TGF-beta is very interesting, because it can induce dormancy but it can also induce proliferation,” Ritsma said. “It all depends.” For instance, TGF-beta spurs the activity of genes that slow the cell cycle to a crawl so a cell can go dormant. To come back to life, a cell can silence these dormancy-promoting genes by altering TGF-beta again.
But this triumphant reversal can have a dark side. As Ritsma has described, in cancers, some fraction of the malignant cells seem to routinely retreat into dormancy, a condition that makes them resistant to assault by chemotherapy or radiation. It may be that some of those dormant cells later reawaken and return to their frenzied state of division, contributing to recurrences of cancers that had been in remission.
Revival in Fits and Starts
No matter the context or the species, cellular resurrection is an ongoing process of adjustment and adaptation. Cells that recover from different kinds of stasis might seem as robust as before, but their recovery may be far from seamless. In one experiment, Hogan and Holly Tang noticed that some of the cells that returned from the brink of death had glitches in their chromosomes after anastasis. That might happen, Hogan Tang said, because cells incur DNA damage during apoptosis and are not capable of repairing all of it.
Most cells pass through a similarly vulnerable stage as they complete the journey back from dormancy. Because water expands as it freezes, some of the cells in wood frogs suffer burst membranes and DNA mutations despite their arsenal of safeguards. Some dormant cells end up too damaged to be repaired and must be sloughed off, casualties of their own stint in cellular limbo.
By studying the minutiae of how cells revive themselves, and how they weather glitches along the way, researchers like Montell, the Tangs and Ritsma hope to induce similar kinds of resurrection in human cells. “Promoting anastasis in dying neurons and heart cells might help to treat brain injury and heart attack,” Hogan Tang said. Storey is taking cues from recovering wood frog cells as he investigates ways to thaw frozen human organs for transplant without damage. That would allow the organs to be stored indefinitely and shipped to recipients all over the world.
Halting resurrection sequences in cells could prove just as fruitful from a therapeutic perspective. During cancer treatment, for example, many cells enter apoptosis because of chemotherapy’s toxic effects, which is exactly what’s meant to happen. However, the toxins may have no effect on cancer cells that have already turned dormant, and even cells that repair themselves through anastasis may retain permanent genetic damage. Cells in both categories might give rise to new cancers or cause existing ones to recur. Montell’s aim is to determine which molecular signals allow cancer cells to progress through anastasis in hopes of suppressing or promoting these signals when necessary.
Still, biologists do not yet know how to identify which cancer cells may awaken from dormancy and divide out of control — information that may be needed to contain overlooked cancer cells before they pose a real problem. The symphony of cell signals that drive anastasis and other types of recovery must also be further decoded. To make therapeutic anastasis possible, “the most important thing is that we try to identify the anastasis inhibitor and the promoter,” Hogan Tang said. Another unresolved question is how neighboring cells communicate with one another to determine whether each cell will return to life, stay dormant, or proceed through a cell death sequence.
Most studies of cellular resurrection have involved cells considered be in the process of dying or only recently “dead” by the usual criteria; the Yale group’s work on slaughtered pig brains that had been dead and unpreserved for hours was an extreme case. But some researchers are not afraid to go much further: They have attempted to revive cellular components from an extinct animal that died more than 20,000 years ago.
Last year, a team of biologists from Japan’s Kindai University, under the longtime leadership of the biologist Akira Iritani, removed bits of Siberian woolly mammoth from a lab freezer, mashed up the tissue in tiny test tubes, and extracted microscopic bits that contained nuclei — the control centers that animated this extinct mammal’s cells during the last ice age. With a pipette smaller than a sewing needle, the researchers injected the nuclei into mouse egg cells using a technique called nuclear transfer. Then they waited to see whether the hybrid cells would show signs of new life. The scientists sat tensely in front of the microscope’s digital screen, alert to any hint of movement.
Suddenly, in one of the reconstructed cells, ancient mammoth chromosomes swam into view and began to link up with the mouse egg’s spindles, which form the apparatus that normally pulls apart the chromosomes in dividing cells. As the team watched, transfixed, the chromosomes migrated into two distinct clusters. Then a faint round edge appeared around each cluster — curves that suggested the beginnings of two cell nuclei where only one had been before.
The mammoth cell nuclei did not progress any further toward division, perhaps because of damage their DNA had accumulated. The genetic engineer Kei Miyamoto, one of the study’s coauthors, stresses that the alignment of the mammoth chromosomes was highly irregular, and that there is no proof that the curvatures seen around the chromosomes were the edges of incipient nuclei. Still, Miyamoto called the observed activity “a good sign.” The researchers had not returned the 28,000-year-old mammoth cells to life, but against all odds, they had at least kindled some biochemical spark within cell nuclei that had last been animated long before human history began.
We now know that cells can flirt dangerously with the boundary of death — and perhaps even cross it entirely — yet regain their lost function. This flexibility is both deliberate and adaptive; at the cellular level, as in broader life cycles, death and revival must remain in exquisite balance for the whole system to flourish. Understanding how that balance is maintained, and how cells adjust it, may give us the liberating yet fearsome power to tilt it toward life.