How 3D Changes in the Genome Turned Sharks Into Skates
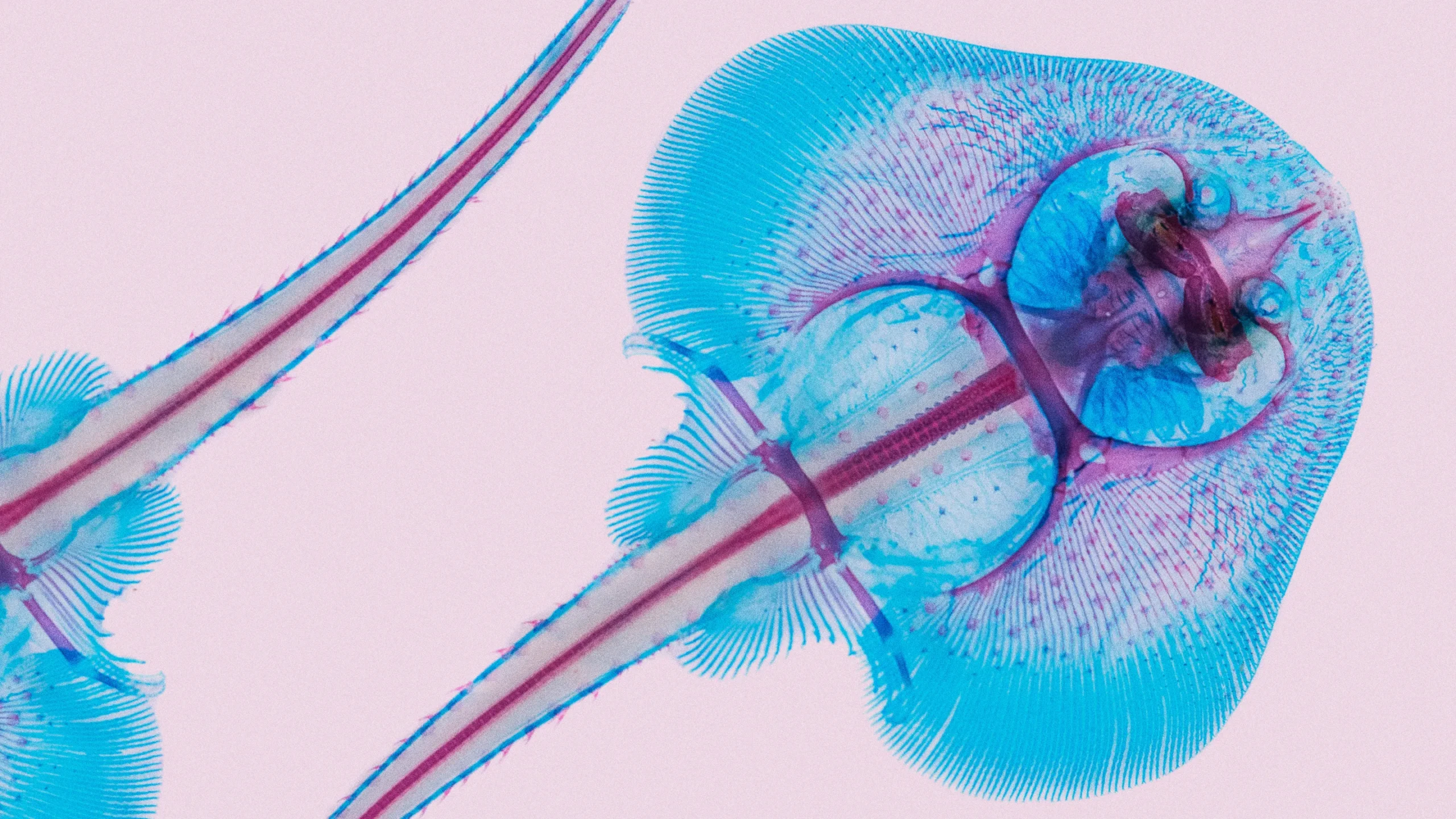
A chemically dyed skate reveals the structural features that contribute to the unusual body plan of this fish.
Teresa Zgoda/Science Source
Introduction
The marine creatures called skates skim along the sea bottom, rippling their winglike pectoral fins to propel themselves and to stir up small creatures hiding in the sand. Their unusual flattened body plan makes them one of the oddest families of fish in the sea, and it seems even odder that they evolved from streamlined, sharklike carnivores that swam about 285 million years ago.
Now researchers have discovered how skates evolved their distinctive profile: Rearrangements in the skate’s DNA sequence altered the 3D structure of its genome and disrupted ancient connections between key developmental genes and the regulatory sequences that governed them. Those changes in turn redrafted the animal’s body plan. The scientists reported their findings in Nature in April.
The discovery solves the mystery of the skates’ evolutionary transformation by pinning it on genetic mechanisms directing development. “The fossil record tells you this change occurred, but how did it actually occur?” said Chris Amemiya, a molecular geneticist at the University of California, Merced who was not involved in the new study. “This is a classic evo-devo question.”
To uncover the origins of skates’ novel body shape, a few years ago the evolutionary genomicist José Luis Gómez-Skarmeta assembled a diverse international team of genomics researchers and evolutionary developmental biologists. A team was needed partly because the first step would be to sequence and assemble the genome of a skate, and compiling the genomes of cartilaginous fish like skates and sharks is forbiddingly hard.
“They’re really difficult to put together, because they’re huge — often bigger than the human genome,” said Mélanie Debiais-Thibaud, an evolutionary developmental geneticist at the University of Montpellier in France who was not involved in the work.
For their work, the team selected the little skate (Leucoraja erinacea), which is easily collected along the Atlantic coast of North America. It can also be reared in a laboratory, which made it possible to run developmental and functional experiments on the animals as part of the project.
By comparing the genome of the little skate to the genomes of other vertebrates, the researchers determined that the skate genome has generally remained very similar to that of their vertebrate ancestors at the sequence level. However, there were a few notable rearrangements that would have affected the genome’s 3D structure. In the DNA of individuals, such rearrangements can cause diseases by throwing off gene regulation. The discovery led the researchers to wonder whether the rearrangements in skates might have similarly disrupted the original genetic instructions for their body plan.
Breaking Down the Boundaries
If you look at the DNA sequence of a chromosome, the genes in it can seem surprisingly far away from the short “enhancer” sequences that regulate the activity of those genes. In practice, though, because of how the DNA in a cell nucleus coils, folds and loops back on itself, they’re often not far apart at all.
In vertebrates, sets of functionally related genes and their enhancers are physically grouped together in three dimensions in units called topologically associating domains, or TADs. Boundary regions help to ensure that enhancers only act on genes in the same TAD.
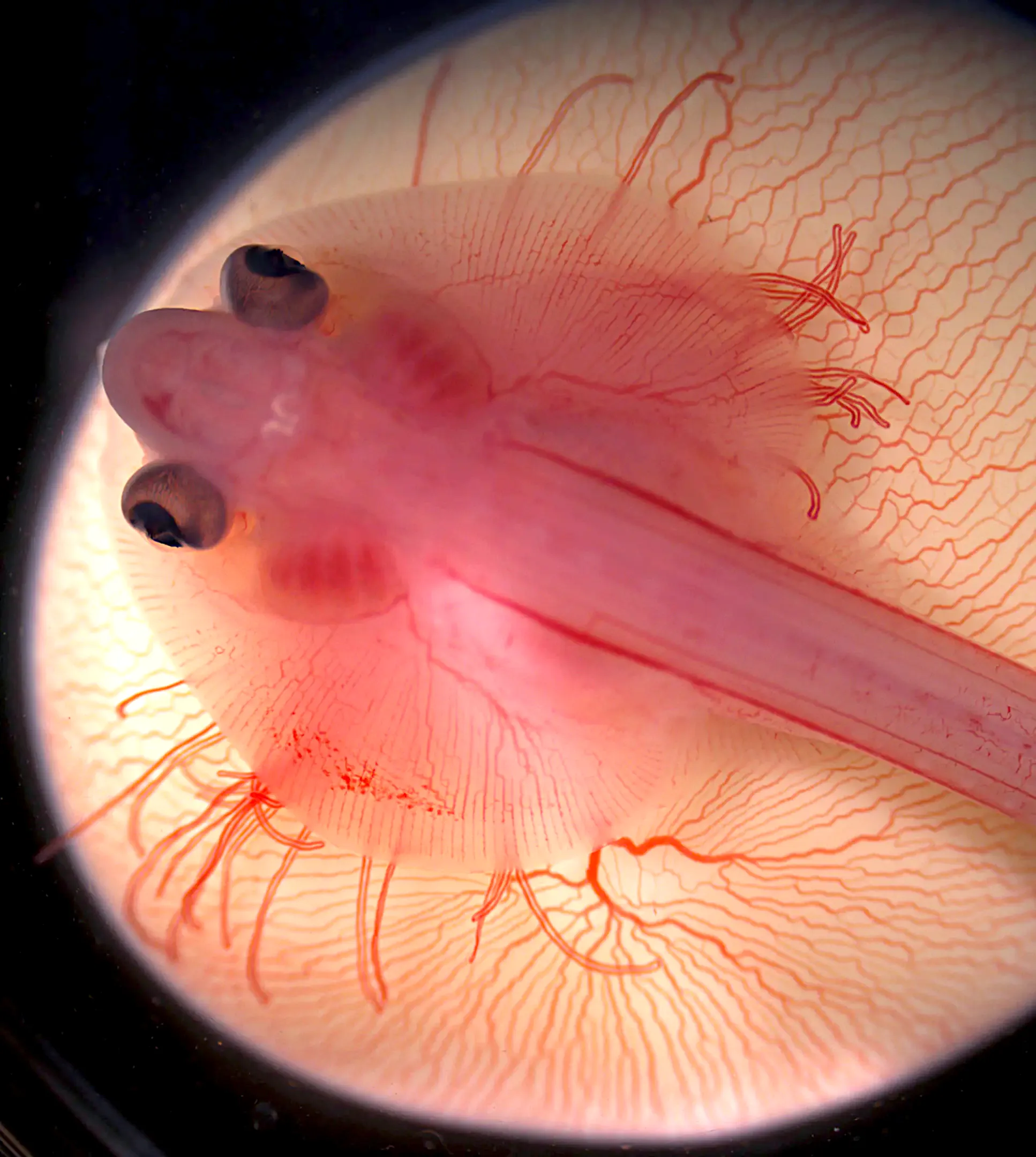
A skate embryo on its yolk sac. Alterations in its developmental program cause the front edge of its pectoral fins to extend forward and fuse with the head.
Mary Colasanto and Emily Mis, Embryology Course, Marine Biological Laboratory (MBL)
However, when major genome rearrangements occur — like the ones that the team was seeing in the skate’s DNA — boundaries can be lost, and the relative positions of genes on chromosomes can change. As a result, “some enhancers can provide instructions to the wrong gene,” explained Dario Lupiáñez, an evolutionary biologist at the Max Delbrück Center in Berlin and one of the senior authors of the study.
It seemed possible that the changes in the 3D architecture of the skate genome might have disrupted the ancient blocks of genes the skates inherited from their sharklike ancestors, affecting the genes’ function. “We were trying to look at whether some genome rearrangements in the little skate actually break these blocks,” said Ferdinand Marlétaz, a genomicist at University College London and co-first author of the study.
The researchers identified genome rearrangements in the little skate that were not present in any other vertebrates. Then they narrowed their focus to the changes that seemed most likely to affect the integrity of the TADs, based on the genome sequences.
The effort led them to a rearrangement that they predicted would eliminate the boundary of a TAD that regulates a developmental system called the planar cell polarity (PCP) pathway. They hadn’t expected that: Nothing about the known functions of the PCP pathway immediately suggested that it would regulate fin development. Mostly, it establishes the shape and orientation of cells in embryos.
A New Genetic Neighborhood
To test out the potential impact of the TAD change on fin development, Tetsuya Nakamura, an evolutionary developmental biologist at Rutgers University, exposed little skate embryos to an inhibitor of the PCP pathway. The anterior (front) edge of their fins was strongly altered and did not grow out to join with the head as it normally would. It suggested that the disruption of the ancestral TAD had produced the skate’s distinctive fins by activating PCP genes in a new part of the body.
“This rearrangement of the TAD basically changes the entire environment of the gene and brings new enhancers into the vicinity of the gene,” Lupiáñez said.
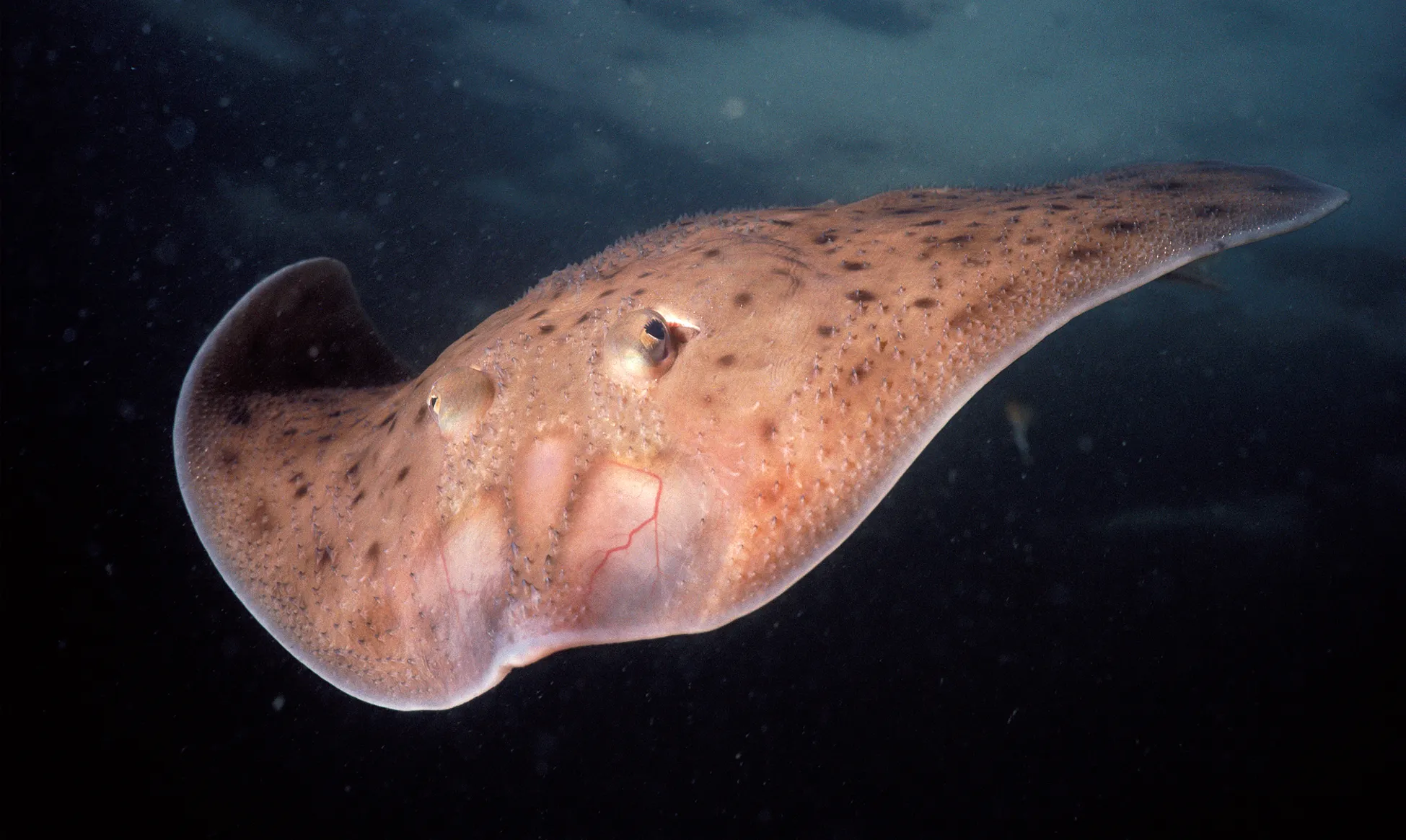
The expanded fins and flattened shape of the skate enabled it to occupy a new environmental niche, hunting near the sea bottom.
Jeff Rotman/Science Source
But that wasn’t the only relevant genome change that the researchers found. They also identified a mutation in an enhancer that regulates the expression of some genes in the developmentally important hox group. Hox genes specify the general body plan in all bilaterally symmetrical animals. One subset of them, the hoxa gene cluster, is usually expressed only in the posterior (back) edges of the developing fins and in limbs, where it specifies the formation of digits.
In the little skate, the hoxa genes were active in both the posterior and the anterior parts of the fin. It was as if the growth zone along the back of the fin had been duplicated along the front, so that the animal made a new set of structures on the anterior of the fin that was symmetrical with structures on the posterior, Debiais-Thibaud said.
Nakamura showed that the skate’s mutated enhancer was causing this new hoxa expression pattern. He combined the skate’s enhancer with a gene for a fluorescent protein and then inserted that gene combination into zebra fish embryos. The fish’s pectoral fins grew abnormally, and fluorescence appeared along both their leading and trailing edges, which showed that the skate’s enhancer was driving hoxa expression in both parts of the fin. When Nakamura repeated the experiment with an enhancer from a shark, the fin growth was unaffected and the fluorescence was limited to the posterior.
“So now we are thinking that the genetic mutations occurred specifically in the skate enhancer, and that can drive unique hox gene expression in skate fins,” Nakamura said.
Shaped for New Ways of Life
In the picture of skate evolution that the researchers have reconstructed, at some point after the skate lineage diverged from sharks, they acquired a mutation in an enhancer that made their hoxa genes active in both the front and the back of their pectoral fins. And within the new tissues growing along the anterior of the fin, genome rearrangements caused the PCP pathway to be activated by enhancers in a different TAD, which had the further effect of making the fin extend forward and fuse with the animal’s head.
“By forming the winglike structure, [the skates] are able now to inhabit a whole different ecological niche, the bottom of the ocean,” Amemiya explained.
Stingrays, mantas and other rays are closely related to skates (they are all classified as “batoid” fishes), and their similarly pancaked shape is probably due to the same genome rearrangements. The rays, however, have also modified their winglike fins in ways that basically allow them to fly through the water. “The skates have these undulations of the fin and stay on the bottom, but manta rays can come to the surface and have a whole different way of locomotion,” Amemiya said.
Although evolutionary developmental biologists have previously speculated that these changes in the 3D architecture of a genome might be possible, this is probably one of the first papers to clearly link them to fairly big changes in body shape, Marlétaz said.
Lupiáñez also believes the findings have significance that reaches far beyond an understanding of skates. “This is a completely new way to think about evolution,” he said. Structural rearrangements “can cause a gene to be activated in a place where it should not be.” He added: “This can be a mechanism of disease, but it can also serve as a driver of evolution.”