The Shape-Shifting Army Inside Your Cells
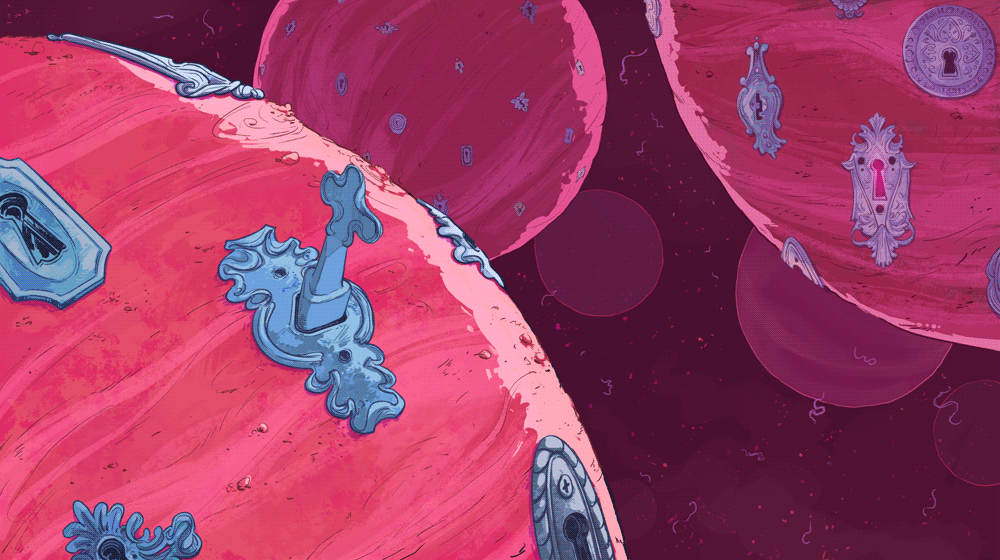
Ricardo Bessa for Quanta Magazine
Introduction
Structure equals function: If there’s one thing we all learned about proteins in high school biology, that would be it. According to the textbook story of the cell, a protein’s three-dimensional shape determines what it does — drive chemical reactions, pass signals up and down the cell’s information superhighway, or maybe hang molecular tags onto DNA. For more than a century, biologists have thought that the proteins carrying out these functions are like rigid cogs in the cell’s machinery.
Of course, exceptions would occasionally crop up. A scientist might bump into a protein that performed its functions perfectly well yet didn’t have rigid structures. Most researchers chalked these cases up to experimental error, or dismissed them as insignificant outliers.
More recently, however, biologists have begun paying attention to these shapeshifters. Their findings are tearing down the structure-function dogma.
Proteins are chains of strung-together amino acids, and recent studies estimate that up to half of the total amino acid sequence that makes up proteins in humans doesn’t fold into a distinct shape. (While some of the proteins that make up this total are unstructured from end to end, others contain long unstructured regions side-by-side with structured ones.) “Partly, people didn’t realize how big that number was, and that’s why they ignored it,” said Julie Forman-Kay, a biochemist at the Hospital for Sick Children and the University of Toronto. “And partly they just didn’t know what to think of it.”
This fluidity — dubbed “intrinsic disorder” — endows proteins with a set of superpowers that structured proteins don’t have. Folded proteins tend to bind to their targets firmly, like a key in a lock, at just one or two spots, but their more stretched-out wiggly cousins are like molecular Velcro, attaching lightly at multiple locations and releasing with ease. This quick-on-quick-off binding’s effect in the cell is huge: It allows intrinsically disordered proteins — or IDPs, for short — to receive and respond to a slew of molecular messages simultaneously or in rapid succession, essentially positioning them to serve as cellular messaging hubs, integrating these multiple signals and switching them on and off in response to changes in the cell’s environment and to keep cellular processes ticking along as they should.
Researchers are just beginning to understand how this paradigm shift will change what we know about the goings-on in the cell. Yet already, IDPs appear to have their paws in a lot of biological processes. Through their signaling prowess, IDPs help regulate the gas and brake pedals for producing proteins from the DNA code, according to evidence that has accumulated over the past decade, as well as the process by which cells divide. IDPs may also provide cues that allow cells to take on traits specific to different tissues or parts of the body. In other words, they may somehow help make a blood cell a blood cell and a muscle cell a muscle cell. Biologists are also finding that many disordered proteins are involved in neurodegenerative disorders, cancers and other diseases.
“The key now is that we need to understand how these proteins are functioning in biology,” said Peter Wright, a structural biologist at the Scripps Research Institute in La Jolla, California. In response to the recent revelations, an international group of researchers has launched a project called the Human Dark Proteome Initiative to study how disordered proteins cause disease. Scientists know that they still have much to learn about what these shape-shifters are up to. “It’s a re-envisioning of cell biology,” said Madan Babu, a molecular biologist at the University of Cambridge.
Outside the Fold
Wright and his close collaborator Jane Dyson first recognized the importance of IDPs more than two decades ago. A researcher in Wright’s lab at the time, Richard Kriwacki, kept coming up with weird results while trying to determine the structure of a protein called p21, which helps regulate how cells divide. “You’ve messed up the prep, Rich — try again,” Wright told him. After the third run, though, they figured the results must be true. On its own, p21 seemed to float formlessly, but when it found the protein to which it normally binds it jumped to attention, taking on a firm shape. The resulting 1996 paper was one of the first to directly challenge the structure-function paradigm.
Around the time Kriwacki did his experiments, some 1,200 miles north at Washington State University, the biochemist A. Keith Dunker was listening to a talk by a former student named Charles Kissinger. The speaker woefully described his difficulties determining the structure of an immune system protein called calcineurin: Do what he might, one very large region of the protein simply refused to come into focus. Kissinger realized that this segment must be unstructured, yet he knew it contained the control center for some of the protein’s most important duties, such as regulating its interactions with other molecules and switching its own activity on and off. The talk reminded Dunker of his own research on a viral protein that had a sneaky way of infecting cells: Folded up, it was too big to pass through the cell’s membrane, but it made its way in by stretching out limp and floppy like a piece of string and slithering through.
This got Dunker thinking — could there be something about the very nature of disorder that facilitates these varying functions? “It was like the proverbial cartoon, with the lightbulb going off,” he recalled. “Seriously, that afternoon I totally changed my research.”
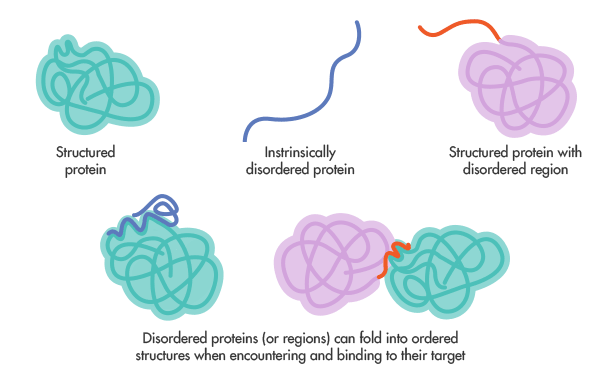
Lucy Reading-Ikkanda/Quanta Magazine
He began to search through the scientific literature for more examples of disordered proteins and quickly found 15 or 20 of them, all described as one-off oddities. He and his collaborators then developed a computer program that mapped the sequences of amino acids that formed each protein and compared them with the sequences of structured proteins. Normally, proteins fold because some amino acids do everything they can to avoid contact with the water-based fluid in the cell. Just as oil balls up in water, hydrophobic regions of a protein clump together for protection, and the parts of the protein that don’t mind water fold over them. Dunker’s analysis revealed that disordered proteins had very different sequences of amino acids than structured ones. When he looked for these differences in databases of sequenced proteins, a surprisingly high number of disordered suspects popped out. Even more interesting, a higher percentage of the protein sequence is disordered in more complex organisms — for example, about 20 percent of the amino acids in the bacterium Escherichia coli are disordered, but the percentage is at least twice as high in humans.
Biologists on the whole, though, remained unconvinced that IDPs did anything interesting or important. Many argued that while proteins might unfold in an artificial laboratory setting, they must be taking on shape when inside a cell. And even if they are able to flop around a bit in their natural states within the cell, surely they snap into structured positions when they encounter their binding partners, just as Kriwacki’s p21 did. The skeptics thought that any disordered proteins had to be one-offs — outliers — and that the classical structure-function dogma held.
“Our answer to that was: It’s not true,” said Wright.
Structureless Function
Protein disorder occurs along a continuum. At one end of the spectrum lie proteins like p21, which fold on contact with other proteins. At the other end are ones that remain limp and floppy, like wet noodle strands, never taking on a shape. Researchers still don’t know how this range corresponds to their versatile functions, but being more like a string than like a lump with keyholes means that a protein can make many contacts with other molecules to regulate the network of signals that drives the cell. “You have all these on-off switches for all kinds of functions,” said Dunker.
But even though IDPs in multicelled organisms make up 30 to 50 percent — depending on the organism — of the proteins that genes are able to make, it turns out that at any given moment, they exist in the cell in only tiny amounts. Babu made this discovery in 2008, after a researcher in his lab raised a niggling question: If these unfolded proteins were in fact so common, and if many of them floated around the cell like limp spaghetti, why weren’t they getting all tangled up, or causing trouble in the cell by tangling up other molecules? When they examined a database of around 5,000 human proteins, they found that most unstructured proteins were expressed in small quantities and quickly destroyed after they had done their job.
The reason cells regulate their production so tightly and make sure they turn over so quickly is that IDPs pack a huge punch, Babu said. Having too many would be like having a glut of upper management — with too many people shouting commands, productivity grinds to a halt. Extend that logic to a cell, though, and things can get ugly: Because IDPs regulate how different components of the cell communicate with one another, having extra copies floating around could leave them sending signals that shouldn’t get sent. “These proteins are so dangerous that you can’t afford not to regulate them,” Babu said.
Babu’s study was the first to reveal a biological principle for how disordered proteins are regulated in the cell. The following year, a paper by Ben Lehner’s group at the Center for Genomic Regulation in Barcelona, Spain, drove the point home: When a cell produces too many of these proteins, they found, it dies. By demonstrating the biological importance of disorder, this pair of papers put the field on the map, said Kriwacki. “A lot of biologists saw that intrinsically disordered proteins are really important — they are not just a figment of the imagination of a group of structural biologists.”
It’s no surprise that IDPs’ significance was overlooked until then, because until recently, most methods used to study proteins would have missed them, Babu said. “It’s like the classic paradigm of looking for the key under the lampshade.”
The most popular approach for determining the shape of proteins was a technique developed in the 1960s called protein crystallography. Protein crystallography involves separating out a pure sample of the protein, coaxing the protein to crystallize, then shooting X-rays at the crystal and mapping where the light bounces off. The problem is, because they have no fixed shape, IDPs don’t crystallize. That left researchers ignoring proteins that were disordered entirely, or finding ways to chop out the disordered parts. “Most crystallographers, including myself, considered this stuff junk — they were things you had to get rid of to obtain the crystals,” said James Hurley at the University of California, Berkeley. “At most we considered them as linkers that connected the interesting bits.”
For him the turning point came five years ago, when a colleague showed him data suggesting that some disordered proteins can form liquid droplets that briefly exist suspended in the fluid of the cell. Researchers still don’t know exactly how or why this process occurs, but some speculate that it brings molecules together for signaling. “At that point I realized this was going to be big,” Hurley said.
Hurley’s own expertise is autophagy, a form of molecular self-cannibalization that was the subject of the 2016 Nobel Prize in Physiology or Medicine. In the past couple of years, he and others found that two disordered proteins help initiate the process. Hurley speculates that these disordered regions act like a weak glue, creating just the right level of cohesion — not too rigid and not too loose — to bring together the molecular components needed for autophagy.
As they continue to explore what disordered proteins do inside the cell, researchers are also pursuing basic questions about how disordered proteins work. If a protein has both disordered and ordered regions, how do the two interact? How did the evolution of disordered proteins differ from that of folded ones? Also, how do molecules figure out where to attach on disordered proteins? Even though both computer analysis and experimental lab tools for probing IDPs have improved over the past five years, studying them directly in a living cell remains a challenge, Wright said.
Researchers also want to explore how disordered proteins contribute to disease. Most drugs are designed to interfere with a specific disease pathway by elbowing their way into important spots inside the cell. But researchers have only begun to target IDPs.
This article was reprinted on ScientificAmerican.com.