How Genetic Surprises Complicate the Old Doctrine of DNA
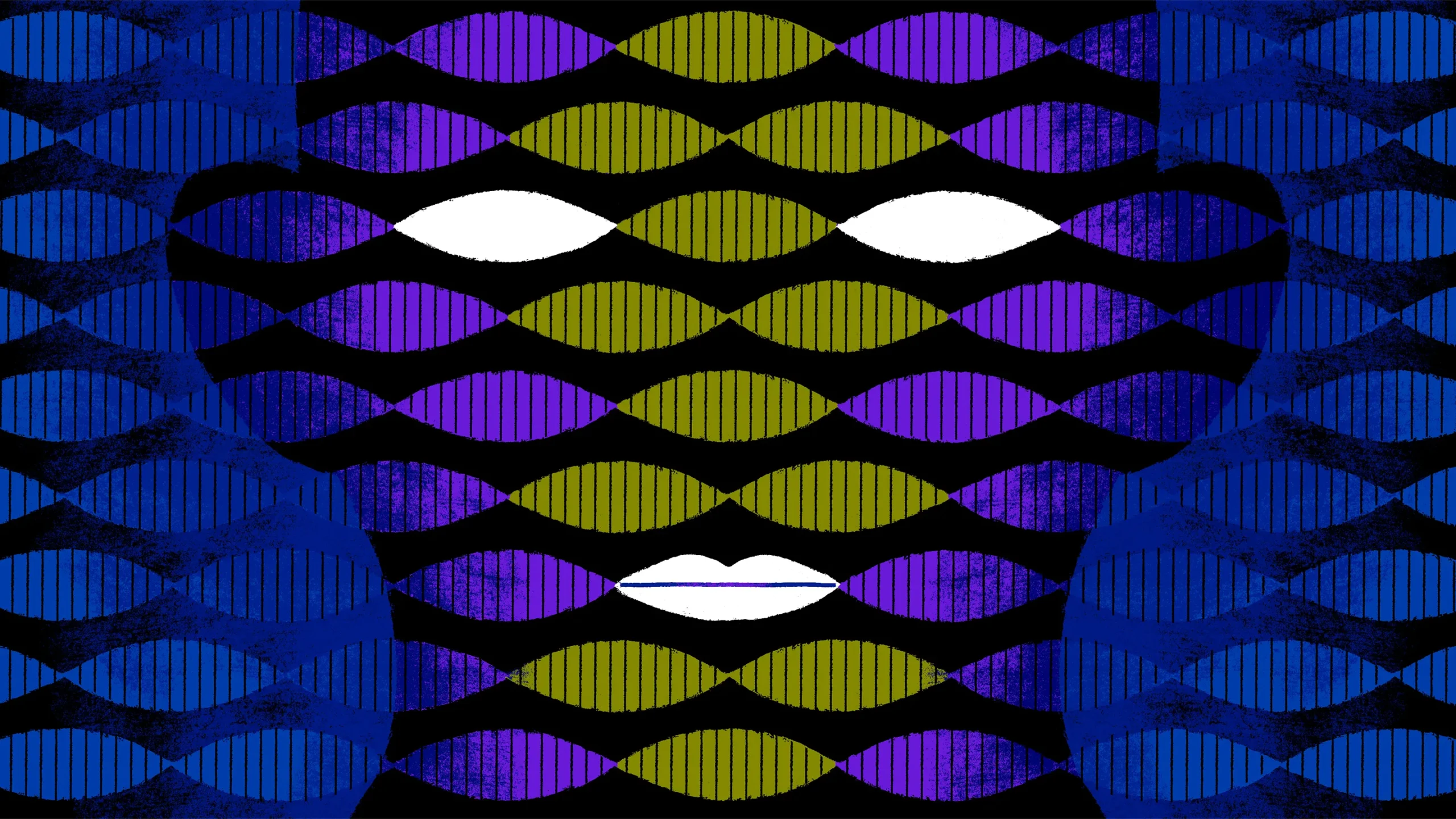
James O’Brien for Quanta Magazine
Introduction
Does an unseen force lurk within genetics? Biologists have made enormous strides over the past 100 years in understanding the role of the millions of parcels that convey our genetic information — DNA, RNA and proteins. But they have also learned about undetectable interactions between these biochemical agents, hiding in their midst like ghosts in the machine, complicating our quest to learn the secrets of life, one gene at a time. These interactions all fit under the umbrella of “epistasis” — not exactly a new idea in biology, but one whose influence and importance are only now being fully appreciated.
Geneticist Daniel Weinreich and colleagues suggested that epistasis is akin to the “surprise” at the effects of mutations when they combine, given what we know about them individually. Whenever a life form’s observable attributes differ from what the DNA would lead you to expect, epistasis may be to blame. Imagine that you know of two hypothetical mutations associated with a flower stem that’s ordinarily 40 centimeters long: Mutation A is associated with a long stem (say, 50 centimeters), and mutation B confers a short stem (30 centimeters). You might expect the mutations to cancel each other out, leaving a flower with a normal-length stem. Or perhaps the combination of mutations adds their independent lengths together, resulting in an extra-long stem (80 centimeters). But instead they end up producing an extra-short stem (10 centimeters). Geneticists would say that there is a nonlinear relationship between the effects of mutations A and B, which gives you the surprising outcome. This is a signature of epistasis.
The flower-stem example represents one of the simplest cases of epistasis, where the nonlinear interaction manifests between two genes or mutations. And there are cases in nature that aren’t so different from this hypothetical one (such as the pigmentation of pigeons, where three genes are responsible). But what about the rest of nature? The human genome, at last count, has roughly 20,000 genes. The genome of the domestic apple (Malus domestica) has over 57,000. All these different genes mean many possible epistatic interactions.
Epistasis can even occur in viruses, which often have relatively tiny genomes, many with fewer than a dozen genes. For example, the HIV virus can develop mutations that make it drug resistant. But these mutations can depend profoundly on the genetic background of the specific form of HIV. To identify the key mutations, you need to know what other mutations are in its genome. With this emphasis on context, epistasis modifies the grammar and logic of molecular biology: It would be easier if “mutations for drug resistance” really were just mutations for drug resistance, no matter what.
This is, in a way, a familiar idea. For example, I teach students in my evolutionary theory course that master chefs are experts at understanding and predicting epistatic effects. The specific challenge of cooking dishes with sophisticated recipes, or of being able to improvise new ones on the fly, is in understanding that ingredients can interact in surprising ways. And so there’s a long tradition of fields that address why the whole can be more than — or at least different from — the sum of its parts.
Indeed, the first references to epistasis came shortly after the rediscovery of Gregor Mendel’s experiments at the turn of the 20th century, which established our modern ideas of heredity and genes. Despite the Mendelian assumption that genes and mutations often act independently in crafting traits, scientists soon observed several counterexamples. In 1909, William Bateson introduced the word epistasis — from the Greek for “standing upon” — while trying to explain the repressive effects of some mutations on the effects of others.
But while the concept was developed long ago, it has taken almost a century for the idea of epistasis to rise to prominence. Partly that’s because it makes things harder for biologists. Its focus on genetic context means that there can be no “gene for mutant powers,” as in our favorite comic books — there is only a “gene that confers mutant powers when in a genome of a certain kind, and/or in the presence of other gene variants 1, 2 or 2,578 (perhaps in a specific combination).” That hypothetical comic book isn’t easy to read or write.
Some biologists remain so opposed to the idea that they don’t believe it’s worthwhile to focus on epistasis. They might object on technical grounds, saying, “Sure, it’s real, but single genes and mutations still matter!” These folks aren’t lying. We know of thousands of single genes or mutations that have a reliably large effect, across many experiments, independent (we think) of the genetic context. That is, there are many important genetic stories to tell without epistasis. Sometimes there are no ghosts.
But part of the opposition can also be charitably characterized as philosophical. This school says that if we can only know how a gene’s mutation works in the apple genome by understanding how it depends on the other 56,999 genes, then that introduces an astronomical number of possibilities — too many to test rigorously. To put it differently, a full embrace of epistasis can feel almost nihilistic. Predicting how genes will work might be tricky, but we can’t set the bar so high that it requires knowing everything about every gene (and mutation). Such a reality would make many of our efforts hopelessly messy. Alas, the only reasonable response to this objection is a common one in science: It is not nature’s job to make itself easy to study, or to submit to our assumptions. Life is complex. We must let it be.
Thankfully, modern breakthroughs in the study of epistasis have worked to partly demystify it. For example, in 2017 the statistician Lorin Crawford and colleagues pioneered a method (known as MAPIT) to measure epistatic interactions between mutations in large sets of data spanning a species’ entire genome — effectively plotting and measuring possible epistatic effects among existing genes and mutations in large genomes. Approaches like this allow us to identify and measure how these ghosts manifest across the genome, helping us understand where human traits come from, including those associated with disease risk.
Other breakthroughs live at the level of proteins: New methods allow us to measure thousands of variants of a single protein, allowing us to see how epistasis manifests in important proteins, such as in the virus that causes Covid-19.
Further, a new idea called global epistasis suggests that the ghost might not be so ghostly. With our hypothetical flower-stem mutations A and B, a global epistasis approach would suggest that the effects of adding mutation B to any genome (whether or not it contains mutation A) will follow a set pattern. Perhaps mutation B acts like a negative amplifier of other mutations, and when in the presence of a mutation that confers a long stem, it reverses the effect. These kinds of patterns have already been observed in several systems independently. How widespread this global epistasis is, and to which systems it applies, is still the object of current research. But it is exciting to know that there might be a way to predict the surprise.
The “ghost in the machine” metaphor was originally used to discuss the mind-body duality problem, the distinction between the nonphysical mind and the mechanical body it controls. It has often been invoked to describe the fear that we might not be building what we think we are — whether it’s increasingly intelligent machines or our understanding of subtly interacting genetic codes. And indeed, bioengineering is where epistasis might cause us the most trouble. Anyone who wants to engineer new livestock (or designer babies) with desirable traits, one mutation at a time, will need to contend with the constant specter of unforeseen consequences — to say nothing of the enormous ethical issues.
These ghosts make the work of geneticists that much more challenging, true. But they also make the biological world that much more fantastic.