How Will the Universe End?
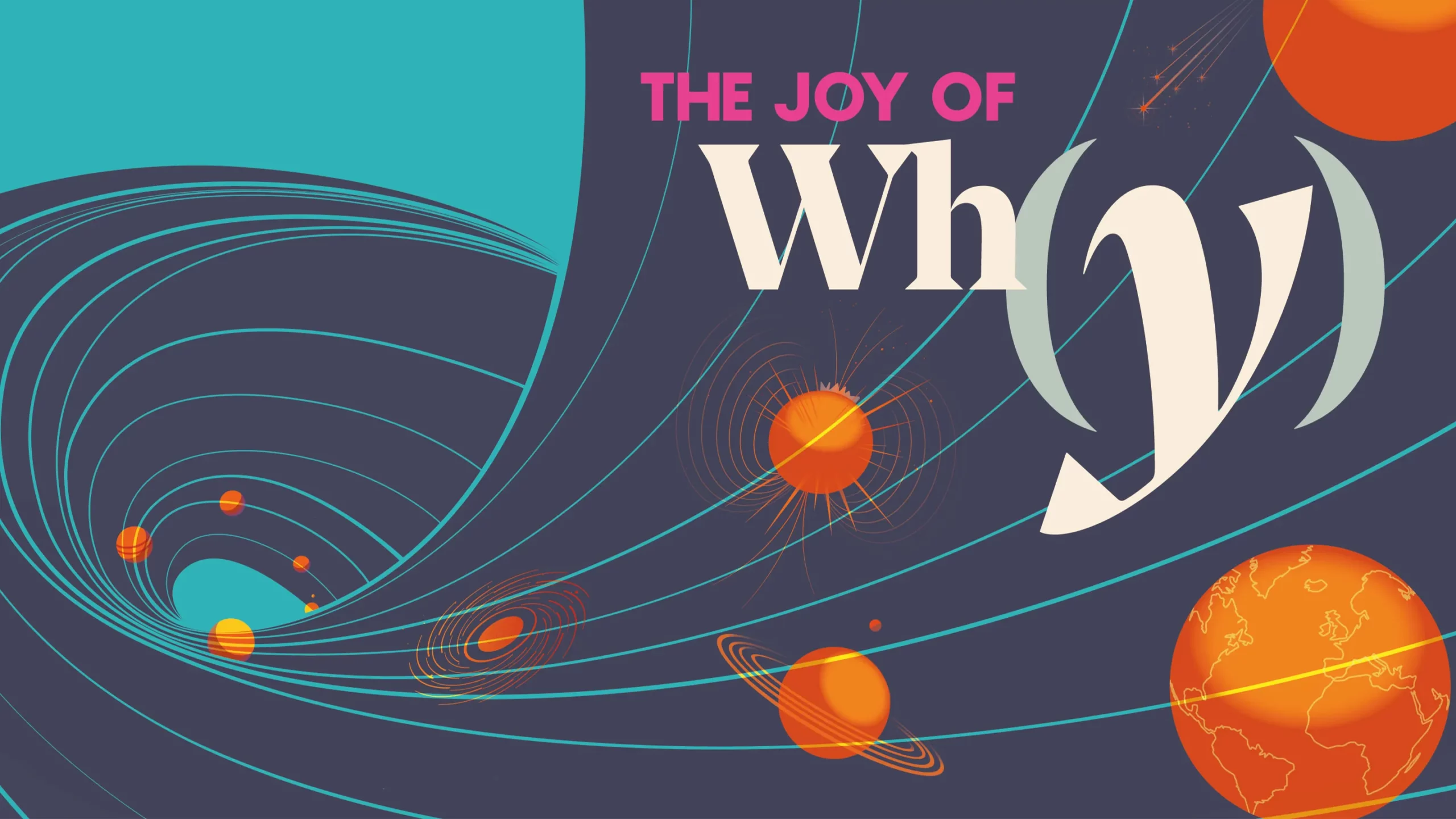
Peter Greenwood for Quanta Magazine
Introduction
Our universe has a beginning. And someday, it will have an end too — but which one? As the cosmos expands and the stars and galaxies grow dim, will everything slowly become colder and more isolated? Could the dark energy that’s accelerating the expansion of the universe eventually rend apart spacetime? Would it be possible for our world and the rest of the universe to one day just cease to exist without warning? In this episode, Steven Strogatz discusses the ultimate grand finale with Katie Mack, a theoretical cosmologist at the Perimeter Institute for Theoretical Physics in Waterloo, Canada. Mack is also the author of The End of Everything (Astrophysically Speaking), published in August 2020, in which she described the five scenarios that scientists have identified for how the universe might end.
Listen on Apple Podcasts, Spotify, Google Podcasts, Stitcher, TuneIn or your favorite podcasting app, or you can stream it from Quanta.
Transcript
Steven Strogatz (00:03): I’m Steve Strogatz, and this is The Joy of Why, a podcast from Quanta Magazine that takes you into some of the biggest unanswered questions in math and science today. In this episode, we’re going to ask, how will it all end?
(00:18) Imagine you’re walking along one day in the city. You’re weaving in and out of other pedestrians walking on the sidewalk. You hear cars honking, quiet conversations seeping out of coffee shops nearby. This is our everyday world as we know it. But what happens if one day that world simply implodes and ceases to exist? What would it be like if everything suddenly came to an end? We do know that stars, including our own sun, have a limited lifespan. They’ll burn out someday, even if it’s not in our lifetime. But what about our galaxy? Or the entire universe? What will the end of everything be like? And how could it happen?
(01:00) This isn’t the makings of a superhero movie. This is the type of theoretical physics that Dr. Katie Mack thinks about a lot. Dr. Mack is a theoretical cosmologist at the Perimeter Institute for Theoretical Physics in Waterloo, Canada, about an hour outside of Toronto. She’s the Stephen Hawking Chair in Cosmology and Science Communication Research, where one of her goals is making physics more accessible to the public. Dr. Mack also is the author of the well-received book, The End of Everything (Astrophysically Speaking), published in August of 2020. It details the five main theories of how scientists think the universe will end. Katie, thanks for joining us today.
Katie Mack (01:47): Thank you very much for having me.
Strogatz (01:48): It’s a real treat for us. Can I start with a personal question? What drew you to this topic — thinking about the end of the universe? Why, why does that grab you?
Mack (01:56): You know, I think that it’s just part of my general curiosity about the cosmos. I grew up thinking a lot about the beginning of the universe, about the Big Bang. You know, all these big questions about where do we come from. And at some point, through my studies in cosmology, I kept coming up against this question of the ending. So I remember reading about the Big Rip — one of these possibilities where the universe sort of rips itself apart — when I was in grad school, and just being fascinated by the concept that the universe could end in this really violent way. And then, as I was continuing to do research in cosmology, I came across vacuum decay — you know, this sort of sudden ending of the universe — and just was fascinated by the concept that the universe could blink out of existence for apparently no reason.
(02:46) And all of these topics just kind of kept coming up in the reading I was doing in my professional work. And I just wanted to explore that some more. And I wanted to tell this story that I think does not get told very often in the sort of public discourse about cosmology. There’s a lot of talk about the beginning, about the Big Bang, but very little about the end.
(03:05) And I think that it’s, it’s just something that’s always been fascinating to me every time I’ve encountered it. Just seeing the discussions around how the ultimate evolution of our universe could complete and what that says about what’s happening now. About the structure of the cosmos, about the overall format of existence. It’s a fascinating question to me.
Strogatz (03:27): Yeah, I mean, it’s — I think it’s pretty natural to wonder about. I think most of us who have some interest in science or just big questions about life do wonder about it.
(03:38) Here’s one that I think we should probably start with: the heat death, the scenario that we call the heat death of the universe, that’s been around for a long time. Tell us about that one, because I understand that you think that may be the most likely one.
Mack (03:50): Yeah, so the heat death is the one that is considered to be most accepted in physics. It’s sometimes called the Big Freeze, colloquially. The idea behind the heat death is, we know the universe is expanding, and we know the expansion is accelerating. So the galaxies that are out in the distant universe, they’re getting farther apart from us. They’re getting farther apart from each other. And this expansion is continuing, and it’s getting faster over time. We don’t know why it’s accelerating — I’ll just point that out. At the moment, it’s [thought to be] due to something we call dark energy. We don’t know what dark energy is, but it’s something that’s making the universe expand faster.
(04:23) Our ideas about dark energy include the possibility that dark energy is just a sort of property of the universe called a cosmological constant, where every little bit of space has a kind of stretchiness just built into it. And as we have more space, as the universe expands, we also have more stretchiness, because we have more of that dark energy, more of that cosmological constant. And so the universe just keeps expanding and expanding and expanding.
(04:48) And if that’s the case, if that’s really what’s going to happen, then what you get is, you get every galaxy or every cluster of galaxies gets more and more isolated from all of the others, and the universe gets more and more empty, more and more diffuse, colder over time. Because, you know, we know that in the very beginning, the universe was very hot and dense. It’s been expanding ever since. It’s cooling, it’s getting more diffuse. So that continues sort of indefinitely. And as that happens, if you’re in a galaxy that’s suddenly isolated because all of the other galaxies are so far away, then there’s no interactions, no galaxies coming in and bringing new gas to form new stars. You as a galaxy kind of burn up all the stars that you have. You burn through all the hydrogen, so you can’t make any new stars. The stars die and burn out and go dark.
(05:36) There’s a bunch of black holes. Eventually, if you leave a black hole alone long enough, it’ll kind of radiate away its energy — the black holes evaporate, everything decays into this disordered energy. So everything that was in this galaxy radiates away. The matter decays and falls apart. And you’d have just this disordered energy, just sort of the waste heat, if you think about that way, of all of the things that existed.
(06:01) And when you get to the stage where everything is decayed away, you reach what’s called maximum entropy. So the second law of thermodynamics tells us that entropy or disorder increases into the future. And you know, [for the] same reason you can’t have a perpetual motion machine, because if you try and get something spinning forever, it’ll break down, it’ll lose some energy to friction and heat, and it’ll fall apart. Similarly, in the universe, everything kind of decays into that waste heat. And that’s why it’s called the heat death. It’s that you have everything to have decaying into disordered energy, and you reach this maximum entropy state where no more disorder can happen, where everything is just kind of fully meaningless. Essentially, it’s fully, fully structureless.
(06:49) That’s the ultimate heat death of the universe. And people do think of it as a depressing way to go, because you end up with everything is very cold and dark and empty and isolated, and just decaying away forever.
Strogatz (07:03): I see why you give it the name Big Freeze, because heat death makes it sound like it’s going to be hot. Whereas if I’m hearing you right, this will be kind of tepid or worse.
Mack (07:11): Exactly. Yeah. And in this case, “heat” is kind of the technical, physics sense of the word where sort of this waste heat of all of creation.
(07:19) But the bright side is that it takes a really long time for that to happen. So it won’t be until about 100 billion years from now until we can’t see other galaxies, because they’re too far away and moving away too quickly. So you know, and that some of the least massive stars in our galaxy can potentially last a trillion years or so. So we have some time before it gets cold and dark and empty in our universe, if we’re going that way.
Strogatz (07:41): The emptiness is another interesting aspect of this, because of the stretching of space. That not only is it really bland, and homogeneous and disordered, but it’s also very lonesome. Like everything is so spread apart from everything else.
Mack (07:56): Right. And a really interesting aspect of that is that you’ll get to a certain point where we won’t have evidence that other galaxies even exist. There won’t be any direct observational evidence that the Big Bang happened, because we won’t be able to see that expanding universe. And we won’t be able to say, “Well, if the universe is getting bigger now, it must have been smaller in the past.” We won’t be able to see the kind of leftover light from the Big Bang, the cosmic microwave background, that allows us to study the very, very early universe. It’ll be not only a cold and dark and empty universe, it’ll be a universe where there’s very little to learn, because we won’t be able to see things beyond our immediate environments.
Strogatz (08:34): I guess just in case anybody’s confused — I don’t think anyone would be — the reference to “we,” you don’t really mean that, right? We’re not here, we’re not around to see anything at that point. We’re disintegrated too.
Mack (08:45): We’re long gone. I mean, the sun will at some point become so bright that it’ll boil off the oceans of the earth. And that’ll only take about a billion years. So we have, you know, between half a billion and a billion years before the Earth is entirely uninhabitable. So, yeah, this is long past that. Whatever comes after us, or if we manage to create little intelligent machines that can carry on our consciousness or, or if we spread out into the stars and you know, live in other places and make use of what little energy there is left in these dying stars. At some point, you know, there will be, we’ll run out of things to do because there won’t be enough energy concentrated in the right way to use it.
Strogatz (09:26): Let’s pretend we believe that space and time are quantized like, a la quantum gravity into things at the scale of the Planck length. If there’s only a finite number of space and time parcels, a big number but a finite number, even under the heat death scenario, wouldn’t there be a recurrence where every state will eventually — I mean, under really, really long timescales — come back? It wouldn’t be the end, even after the heat death.
Mack (09:54): I do talk about this in the book in the heat death chapter, the idea of eternal recurrence. Yeah, so there’s one way of looking at the heat death where you’re kind of in this eternal heat death state where entropy is maximized. But even in a maximum entropy state, you can have random fluctuations where something can come together. And there have been interesting calculations where you can calculate, based on a fully homogenous disordered universe, how long it will take, for a grand piano to randomly assemble itself in the middle of the universe, just in the middle of the void.
(10:29): And it’s a really, really big number, right? But if you have this really eternal state, then that will happen. It’ll happen an infinite number of times on some recurrent timescale. And you can extend that and say, well, if a grand piano can assemble itself, so can the Earth, so can the galaxy, so can the entirety of any state that has ever existed in the universe. So when you get to that point, you can say, well, this moment, right now, the specific distribution of atoms and molecules in the universe right now, at this point, it must be possible for that to happen again — on a really, really long timescale, but it must be possible for this to recur. And then the universe will just evolve toward the death again, from this point.
(11:13) And so you get to this idea where every moment that’s ever happened in the history of the universe can happen again, an infinite number of times. And it’s a really mind-bending concept. Now, there are arguments about this in the literature, whether or not this is a sensible calculation to do. But it does kind of bring back — there’s a nightmare scenario that Nietzsche wrote down that was based on this idea. That you, you live the same moment over and over again forever. And wouldn’t that be horrible? And, you know, maybe that is physically possible, maybe that is a thing that can happen. The literature kind of goes back and forth about whether or not you should think about this in this way. But it is interesting. And it also connects to this possibility that, let’s —. If a, if a grand piano can assemble itself in the universe, so can a single brain that thinks it has experienced the entirety of the cosmos? This is called the Boltzmann brain hypothesis.
Strogatz: Oh, I’ve heard of that. I didn’t know what that was. OK, cool.
Mack (12:12): So maybe instead of everything existing, there is a brain that at this moment thinks it’s having this conversation and has lived a whole life in a universe of 13.8 billion years old. And then at some point, that brain is just going to blink out of existence, again, because it was a random collection of particles in an empty post-heat death universe.
Strogatz: OK…
Mack (12:33): So you can do that calculation as well. And if you do that calculation a certain way, you find that that’s much more likely than the universe existing at all.
Strogatz: Uh huh.
Mack (12:42): It’s much more likely to produce a single brain that thinks it’s in the universe than it is to produce, you know, a new Big Bang and then an actual cosmos. But again, there are different ways of calculating it where you get different answers. So that’s another piece of the question of, does it even make sense to do these calculations? And if you do this calculation, you find that we’re more likely to be a random thought in a random brain, just existing in the void. It doesn’t tell you necessarily, that’s the likely scenario of the universe, it tells you that these calculations are not useful, and do not really make sense in the context of the cosmos, and something about our assumptions must be off. But how do you deal with this possibility of an infinite universe in which anything could happen an infinite number of times is a really interesting question in cosmology when you get to these really, really huge timescales.
Strogatz (13:36): All right, well, thank you for indulging me on that. OK. But I do want to make sure we get into some of these others.
That was Scenario #1, the heat death, the Big Freeze, and this nice footnote about eternal recurrence in the, in the wild — I don’t want to say paradoxes, but, but really mind-stretching kinds of considerations that it brings up. OK, let’s move on to #2. What’s the Big Rip?
Mack (13:58): So the Big Rip is an idea that comes back to this question of dark energy. We don’t know what it is that making the universe expand faster. We call it “dark” energy because we don’t know what it is. But there’s something that’s accelerating the expansion of the universe. Now, if it’s just a cosmological constant, if it’s just a property of the cosmos, then we know how that goes. You know, it leads us to heat death, where all the galaxies are maximally isolated, and then they fade away.
(14:23): But there are other hypothetical possibilities for dark energy. There are some where instead of being just a constant background in the cosmos, it’s something that is dynamical. It’s something that could change over time. And specifically, you can write down equations for something where it gets more powerful over time. Where whatever this is that’s the kind of stretchiness built into the cosmos, it’s a dynamical field, an energy field, and it gets more powerful over time. And so that it starts stretching the universe faster and faster. Not just causing acceleration but building up within objects.
(14:57) So one thing about a cosmological constant. If a cosmological constant exists, the density of it is constant in the universe. What that means is that if you draw a sphere around a certain region, there’s a certain amount of cosmological constant in that sphere. And even as the universe expands, there’s still the same amount in that sphere, right? The cosmological constant remains the same. In a universe with what we call “phantom” dark energy, the amount of dark energy within that sphere would be increasing over time. If you had a galaxy living in that sphere, for example, and that galaxy’s gravitationally bound and everything’s kind of held together by gravity, in a cosmological constant universe, that’s fine. The orbits don’t change. The galaxy stays as it is. In a universe with phantom dark energy, the amount of stretchiness inside that sphere is building up. The dark energy is building up and it can pull the galaxy apart. It could pull the stars away from the galaxy, it could pull planets away from stars, and it would just build up and build up within objects.
(15:55) So instead of a situation where all the dark energy is doing is just moving distant things away from each other, just kind of creating more empty space, it would actually be stretching things from within. I often tell people, like, “Oh, you know, the universe is expanding, what’s happening is distant galaxies are getting farther apart. But this room isn’t expanding.” In a universe with phantom dark energy, this room would be expanding, eventually.
Strogatz: I see.
Mack (16:19): So what it would do is, it would start by building up on really large scales. So it would pull old galaxy clusters apart. It would pull the stars off the edge of the galaxy. But it would get more and more powerful, so that it would start to pull planets away from stars, start to take moons away from planets and build up within planets and eventually explode a planet itself. And then it kind of gets more and more powerful as it goes farther down and you eventually tear apart molecules, tear apart atoms, and ultimately tear apart the universe itself.
Strogatz (16:50): So is it really the case that under this picture that you described, it’s as though it was descending through the length scales from the biggest down to the smallest. It’s going to go in that sequence?
Mack (17:00): Well, what it is is, it’s getting more powerful. So it’s unbinding the most weakly bound things first, the largest things are most weakly bound. And then as you get to smaller and smaller scales, you’re getting to like atomic binding, nuclear binding. So just stronger bindings.
Strogatz: I see. I see.
Mack: It kind of builds up in that sense.
Strogatz (17:18): Wow, that’s an interesting one, things are getting sort of ripped from within, as opposed to just… Like, I had pictured with the, the heat death and the cosmological constant scenario, almost like when we talk about how the universe is expanding, and people say, “Well, what’s it expanding into?” And then someone says, “No, picture painting dots on the surface of a stretchy rubber balloon,” you know, or like that. This is sort of the cosmological constant. It sounds like the dots on the balloon get farther apart. Those being, say, the galaxies getting farther apart. Is there a picture that replaces the balloon for the Big Rip? It sounds much more violent.
Mack (17:55): Well, when I’m using a balloon metaphor, I usually say, like, imagine, like, little ants on the surface of the moon. And as the balloon gets bigger, the ants get farther apart. But the ants themselves aren’t really paying attention to that. They’re sort of their own little objects. In the Big Rip scenario, it will be more like if you draw a galaxy on the balloon and then expand the balloon. Even the galaxy itself is gonna get bigger in that picture. And so the objects themselves are gonna get larger. And at some point, you get to the point where the balloon itself sort of explodes. You didn’t get to figure out that way.
(18:26) There are issues with the balloon analogy in terms of the details, but that’s kind of a picture you can have.
(18:53): Now, I should say that most cosmologists do not think that the Big Rip is going to happen. It breaks certain rules about energy conditions in the universe. So things that we think should be true about how energy moves through the cosmos, phantom dark energy breaks those rules. And so it’s probably not viable as a scenario. But that said, we can’t entirely rule it out observation, all we can say is that when we look at how the universe is evolving now, we can say that the Big Rip is almost certainly not going to happen within the next, say, 200 billion years. Because you can’t ever say that it’s 100% not going to happen. But based on our measurements, we can put kind of a limit in time and we can say it’s almost certainly not going to happen within a certain timeframe.
Strogatz (19:15): Huh. Well, should we move on to #3? This one I have heard comes about from things that we’ve learned at the Large Hadron Collider and word on the street is that this one might be your favorite, even if you don’t think it’s the most likely. It goes by the name vacuum decay theory.
Mack (19:33): Yeah. So vacuum decay is something I only learned about right around the time that the Large Hadron Collider discovered the Higgs boson. And the reason that I heard about it then is because people started writing papers about vacuum decay in response to the discovery of the Higgs boson. Because the properties of the Higgs boson suggested that vacuum decay might actually be a possibility.
(19:56) The idea behind it is this. It’s quite a technical story, but I’ll try and simplify it. So the idea is that the interesting thing about the Higgs boson is not the particle itself. It’s the fact that that the Higgs boson implies the existence of the Higgs field. Now the Higgs field is a sort of energy field that’s throughout all of space. And essentially, what the Large Hadron Collider did was, it kind of excited that energy field, excited a particle out of that energy field and the particle was the thing that was identified. But it means that there’s this field of energy that exists through the universe. And that energy field has some value. And we call that energy field the Higgs field. And there’s a whole story about how particles interacting with that energy field is how certain particles have mass. And it’s tied into that whole picture.
(20:43) But from a physics point of view, the important thing about the Higgs field is that there was a process that happened in the very, very early universe where the Higgs field changed. So in the very, very early universe the Higgs field had a different value. It’s kind of like it’s a field that has a value kind of like in the sense that like, the temperature in this room has a value everywhere. You can define a temperature field, and it has different values, whether you’re close to the window, close to the door, whatever. The Higgs field would be a field where it has the same value everywhere, but it’s a field with a certain value throughout space. It has some energy associated with it.
(21:15) Now, what value that Higgs field takes has a relationship to how particle physics works in the universe. So in the very, very early universe, the Higgs field was different. The particles interacted with it differently, and there were a different set of particles in the universe. None of them had mass. And there were different interactions in the universe. We had, instead of, you know, electricity and magnetism and the strong and weak nuclear forces, we had a different set of forces. There was a sort of combination of forces that existed, and different particles existed and none of them had mass. And then there was an event called symmetry breaking, where the Higgs field changed, it took on a different value. And when that happened, that allowed for the existence of all of the particles and fuels that we understand now in the universe. So you know, electrons and quarks, and it allowed for the existence of the electromagnetic force and strong and weak nuclear forces. Everything kind of settled into the kind of physics that we experience today. And that was good because that means that we could have atoms and molecules and we could exist.
Strogatz (22:16): I’m sorry, I had to pause there, because that sounded very biblical. “And that was good,” right? That’s what it says, right? “Let there be light. And God saw that it was good.”
Mack (22:26): Well, I mean, in this case, we are very happy that the Higgs field changed, that this symmetry-breaking event occurred because it allowed us to exist. I mean, you can talk about, you know, if it hadn’t happened, we wouldn’t exist to be happy about it. There’s a whole argument there. But in any case, it happened; now we exist.
(22:41) The problem is that when the Higgs boson was discovered, measurements of the mass of the Higgs field, and the masses of other particles, give us hints about what the Higgs field is doing about how the Higgs field has evolved. And those hints seem to point toward the possibility that the Higgs field could change again. That would be really bad in the same way that to the first time the change was good. If it changed again, it would change us into a situation where we cannot exist, where our particles do not hold together. The constants of nature would change. There would be different forces and different particles. It would switch us into what’s called a true vacuum state. I don’t mean “vacuum” in the sense of, like, nothing existing. Vacuum states are different states of how physics works, essentially. So we talk about we’re in a certain vacuum state. There could be a different vacuum state. So if the Higgs field really does have this possibility of changing, then that means the vacuum state that we’re in is called the false vacuum. And the true vacuum would be the vacuum state that the universe would kind of rather be in, that the Higgs field would kind of rather be in. And it would be that eventually, if you wait long enough, the Higgs field will change to that other value, and will sort of evolve into the true vacuum state.
(24:01) And the way it happens is kind of … dramatic. So you can think of it as the universe being kind of metastable, meaning “not entirely stable” in the same way that, like, if you put a coffee cup on the edge of a table, it’s gonna sit there, but something could knock it off, and it could fall down, and it would really rather be on the floor. And you can think of our Higgs field as potentially being in that kind of state, where all that you would need is, in order to shift it into that other state, you need to either disturb the Higgs field directly in the same way that you could, you know, knock a coffee cup off of the table. Or you would just need to rely on the idea that all of these particles and fields are relying on quantum mechanics, the rules of quantum mechanics, and quantum mechanics says that sometimes, sometimes your coffee cup might just fall to the floor anyway, right? The quantum mechanical uncertainty says that every once in a while, if you put a particle on one side of a wall, it’ll just show up on the other side. That’s called quantum tunneling. That is a thing that happens that we observe on the subatomic scale all the time. And that applies to the Higgs field too.
(25:03) And so there’s some kind of decay time associated with the Higgs field in the state where if you leave the Higgs field alone long enough, eventually one bit of that Higgs field somewhere in the universe will quantum tunnel into this other state. And that might not be a problem as a state on the subatomic scale. But unfortunately, if one piece of the Higgs field goes to this new state, goes to the true vacuum, that all of the Higgs field around it also falls to the true vacuum.
Strogatz (24:33): Oh, really? So there’s some kind of chain reaction like it ignites the whole thing.
Mack: Exactly. Exactly.
Strogatz: I don’t know if that’s the right word. But yeah.
Mack (25:35): Yeah, yeah, it would be like, if you had a chain on a table and you — and one link fell off the table, it would pull all the other links down as it falls. And you’d have something like that happening. You’d have this cascade, where as soon as the event happens in one point, it happens all around it, and it would create this bubble of the true vacuum state that would expand through the universe at about the speed of light.
Strogatz: Oh.
Mack (25:58): That’s bad for a couple of reasons. One, is that the kind of edge of the bubble, the bubble wall has some energy associated with it, where if the bubble wall hit you, it would sort of incinerate you immediately. Also, if you pass into the bubble, you’re in this true vacuum state where the laws of physics are different, and your particles don’t hold together anymore. And then furthermore, there was a calculation done in the 1980s that suggested that, once you’re inside the true vacuum state, the, the space there is fundamentally gravitationally unstable. And so you would immediately collapse into a black hole.
Strogatz: Man, you get it from every direction.
Mack (26:34): Exactly, exactly. And so if this occurs, if this quantum event happens at one point in the universe, then that bubble expands at about the speed of light and just destroys everything in the universe. And because it’s happening, it was speed of light, you don’t see it coming. By the time the signal of it gets to you, it’s already on top of you. But on the other hand, you wouldn’t feel it because you know, your nerve impulses don’t travel that fast, you wouldn’t really notice that it happened. But you would just blink out of existence.
Strogatz (27:04): I mean, the speed of light makes it an interesting thing, since the universe is very big, even relative to the speed of light. So it could be happening somewhere far away, 13 billion light years away, no?
Mack (27:16): Sure, sure. It’s certainly true that there are parts of the universe that are being pulled away from us more quickly than the speed of light by the expansion of the universe. And so if the bubble occurs in one of those distant regions, then that bubble will not reach us. But because it’s kind of a random event with the same decay rate everywhere, if a bubble happens really far away, it’s just as likely to happen nearby.
Strogatz: Aha. OK, good point.
Mack (27:40): So fortunately, the decay time that we can estimate from our current data is something like 10 to the power of 100 years. So it’s not something that we think would happen anytime soon. If we do think it’s going to happen, then it’ll be a very, very long time from now almost certainly. But because it’s a quantum event, it’s fundamentally unpredictable exactly when it would happen, the same way that you can’t predict, you know, when a particular atom is going to decay in a radioactive decay process. You can only give a sort of half-life for a chunk of the stuff. Similarly, with the universe, we can’t say with certainty that it’s not going to happen right here, you know, in the next five minutes. We can just say, most likely, in our observable universe, it won’t happen in the next 10 to the power of 100, or 10 to the power of 500 years.
(28:25) The other caveat to keep in mind is that these calculations are based on taking what we know about the Standard Model of particle physics extremely seriously. And the Standard Model of particle physics, which is our sort of understanding of how particles work in this universe, is, we think, incomplete. It doesn’t include dark matter; it doesn’t include dark energy. We’re pretty sure that there are holes in it. And if we really had a more complete picture of particle physics, it might not include the possibility of vacuum decay at all.
Strogatz: OK.
Mack (28:58): So vacuum decay is an idea that comes about when we sort of extrapolate beyond what we think, you know, is the limit of validity of our theories. But it is a fascinating possibility. The reason I enjoy it so much as an idea is that it’s this very, very profound connection between the tiniest scales, the very, very early universe and the destruction of the entire cosmos.
Strogatz (29:21): Nice. Right. I mean, it’s, it’s very…. It’s just, there’s something so fundamental about this mechanism, where the whole laws of physics just change on you in a blink of an eye. But also that what a picture this idea of the, the edge of the vacuum bubble or whatever you called it coming at you…. Yikes.
Mack: Yeah.
Strogatz (29:42): Theory #4, it’s time for theory #4 to step onto the field here. This is the scenario known as the Big Crunch, which certainly sounds violent and interesting. What, what is the Big Crunch?
Mack (29:56): Well, the Big Crunch is an idea that’s really been around quite a long time. It was the idea that was sort of most accepted as likely in sort of the 1960s. The idea behind the Big Crunch is that we observed that the universe is expanding. And there’s the question we have to ask: Is the universe going to continue extending forever? Or will it re-collapse at some point? So we know the universe was small and hot and dense at the very beginning. And it’s been expanding ever since. And there should be some interplay between the expansion and gravity in that whole story, right? So as the galaxies are being pulled apart from each other, by the extension of space, they also have gravity pulling toward each other. And so the existence of matter in the universe should just slow the expansion through the fact that everything is attracted toward everything else.
(30:41) Over the years, there has been an attempt to figure out, is the expansion going to win? Or is gravity going to win? And we now know that the expansion is very likely to win, because we see that the expansion is actually speeding up, because dark energy is making the expansion speed up. And so we don’t see a clear way where the universe could stop and re-collapse. But back in the 1960s, we didn’t know, and the preliminary data seemed to suggest that there was more gravity than expansion in the sense that the universe would stop expanding, and eventually re-collapse.
(31:13) And I should also say that, you know, we don’t think this is a favorite idea now. But because we don’t know what dark energy is, we don’t know for sure that it’s not something that could sort of turn around. You know, we know that it’s causing expansion now. We don’t know that it’s not something that could change, that might be some dynamical field where at some point, it would cause a compression instead of expansion.
(31:34) So we don’t know for sure, but I think it’s the scenario that I find most terrifying, even though in a sense, it may be one of the least likely because it seems to contradict the current data. The idea that the universe could start compressing everything is really, really upsetting. Because, you know, right now we see the galaxies getting farther away. We see the universe sort of cooling and emptying out. If the universe started to contract, then what we would see is, we could see all these distant galaxies kind of rushing toward us. And galaxies would be colliding with each other all the time, but distant galaxies would come toward us and the universe would get very, very dense and crowded.
(32:12) And worse than that, all the radiation in the universe would also be compressed. That means not only would it get hotter, just because more radiation is in a smaller space. But also all of the radiation would be kind of hardened into higher energy radiation, higher frequency radiation. So there’s a process that happens in the universe during expansion called redshift, where radiation is stretched out to longer wavelengths. So you know, visible light becomes infrared, becomes radio. If you had compression, then all of that visible light from all the stars that have ever shown in the universe would start to be compressed into ultraviolet, into x-ray, into gamma ray light. And it would start to just cook the universe in this very profound way.
(32:57) And there was a really fascinating paper from, I think, 1969 by astronomer Martin Rees, where he calculated that in this Big Crunch scenario, at some point, the ambient temperature of space, the radiation in space from just all that starlight being compressed, would be enough to cause thermonuclear reactions along the surfaces of stars, and would cook the stars from the outside in, just from the radiation of space. And you know, at that point, like nothing is survivable. So it’s an idea that I find personally quite upsetting, the idea that we could just be cooked by the radiation of space as the universe is kind of collapsing all around us.
Strogatz (33:38): Well, yeah, interesting that that’s the one that bugs you the most, because I mean, all of them have their own…. You know, like, do you want to go suddenly? Do you want to boil? Do you want to freeze?
Mack (33:49): Right. Right. I mean, none of none of them end well, right? But with the heat death, you have a really long time. So that’s nice. You know, it’s all kind of gentle. With vacuum decay, you don’t see coming. So like, whatever, you don’t even notice.
Strogatz: OK.
Mack (34:04): It’s kind of a non-event, from the perspective of a conscious being. But both the Big Rip and the Big Crunch, you would see coming, and that is quite scary.
Strogatz (34:13): Uh huh. I guess we’re now up to the last one, the Bounce, or what I think I remember as a child used to be called the Pulsating Universe. Is that the same idea?
Mack (34:23) So in this case, I’m kind of lumping a few different ideas into one broad category of cyclic universe or bouncing universe. The idea there is that essentially attempts to explain the very beginning of the universe…. So there are certain aspects of the early universe that are hard to explain in our current cosmology, you know. How did it get set up the way it was? Why is our universe the sort of shape it is, in terms of the sort of shape of space? Why was our universe low enough entropy in the past that the entropy can be increasing into the future to the state where it is now?
(34:54) These are all profound questions about the very beginning. And there have been some attempts to answer these questions by saying, “Well, maybe the beginning wasn’t the beginning. Maybe there was something before the beginning that created the conditions for the universe that exists today.” Those lead to these cyclic cosmologies. Either an idea where there was a previous universe that evolved into the Big Bang that we experienced and then evolves into our current universe. Or simply where you just have a constant cycling of universes, where there was something before us, there will be something after us. And some of those ideas involve kind of compression to the new Big Bang, some involve a sort of heat death, and then a new Big Bang coming out of that. Some are sort of, “there was a previous phase, and that evolves into our phase, but nothing’s going to happen in the future.” So these are all kind of ideas that get picked around for possibilities for either the future of our universe, or the end of a previous universe leading into ours.
Strogatz (35:48): At this point, I guess, I like to put on my … not really my skeptics hat, but my scientist hat. It seems like there’s a lot of science in what you’re saying, in that you’re connecting it to what we know about quantum field theory or about general relativity. But what about observations?
Mack (36:05): Yeah, I mean, so fundamentally, we’re never going to be able to answer with complete certainty the question “how the universe will end?” Because, obviously, if it happens, we are not there to write down the answer. But there are a few different ways we approach this question that fundamentally, what we’re trying to do is extrapolate what we know about the universe now and its evolution from the past into the future. And that’s where you end up with this branching of different possibilities. Because there are several different directions that could go and we could go in the future that are consistent with the evolution of the universe up until now.
(36:37) In terms of observational things that we can learn that can tell us more about which of these paths is more likely, there are a few different ways to approach it. One is to try and understand dark energy. So three of these scenarios are hinging very much on what dark energy is, and how it will act. So if we can figure out is dark energy really a cosmological constant? Or is it something that varies? And that might be an impossible question in and of itself because a cosmological constant is kind of a special case of a broader class of dark energy ideas, where you can never be 100% certain that you’re exactly in that state.
(37:16) It’s a little bit — observationally, it’s hard to be there with complete certainty, but we can get more and more certainty about the behavior of dark energy. And maybe we could find a sort of theoretical basis for dark energy. Maybe there’ll be some experimental result in some other way that will tell us that this is really the answer for what dark energy is. So trying to understand dark energy either through cosmological observations, or through experimental tests that can get to the possible sort of fundamental physics of dark energy. Those are all avenues we can explore and try to distinguish between heat death, Big Rip, Big Crunch — those kinds of ideas that hinge on the expansion dynamics.
(37:55) In terms of something like vacuum decay, if we better understand the Higgs field and its connections to other particles and other fields in particle physics, then we’ll get a better idea of whether or not the Higgs field is even capable of decaying in this way. And whether vacuum decay is a possibility, how the Higgs potential changes at different scales. These are all things that are actively being researched with experiments like the Large Hadron Collider.
(38:22) And then when we’re talking about cyclic universes, there we just really need to understand the beginning, right? If we get more information about the very, very early universe through observations, through sort of clever analysis of early universe data, through looking for things like primordial gravitational waves, and what that might tell us about whether or not cosmic inflation occurred in the beginning, or through a better understanding of the particle theory through things like particle experiments that could tell us if the Standard Model of particle physics is really valid, or what else might be underlying it, if there could be higher dimensions of space? That’s another aspect of this question.
(38:59) So all of those are places we can look for trying to understand if cyclic universes are the right direction to be going. And whether there was something before the Big Bang that set up the conditions for our universe today.
Strogatz (39:11): So it does sound like lots of different avenues within fundamental physics is our best shot here. Let’s just talk about the Webb telescope, because I’m sure a lot of people are thinking about that, since especially what you just mentioned in the last case about the cyclic universe is that it’s so much a question about what’s happening in the early universe. And the Webb telescope tells us something about the early universe, but I’m guessing not early enough. Is that right?
Mack (39:35): Yeah. So, the Webb telescope can tell us a lot about the earliest generation of galaxies. And that’s super exciting for me personally, because as a dark matter researcher, the impact of dark matter on those first galaxies could be really different in different kinds of dark matter models. So there’s a lot we could learn about certain aspects of fundamental physics, about things like dark matter, essentially about dark energy as we observe very distant galaxies. and potentially get just a better measurement of the geometry of the universe as we get more of these galaxies. So we can certainly learn a lot about the galaxies and about the large-scale structure of the universe, we’re gonna get some information from the JWST from those kinds of observations.
Mack (40:15): In terms of the very, very early universe, though, it’s really observations of things like the cosmic microwave background. So this kind of light from the very early universe where the universe was still on fire. But it is still in this sort of hot radiation phase, it was glowing with heat and with radiation from this primordial plasma. And with microwave telescopes, we can see that glow. And that can give us some really important information about the very, very, very early universe.
Strogatz (40:42): What do you think about the field of the study of the end of the universe? Any thoughts about where it’s going to go in the next 10-20 years? Is it just that we’re going to keep plugging away on fundamental physics, and that’s going to be our best hope for really making some progress here?
Mack (40:58): I think that’s true. I think that as we continue to learn more about the fundamental nature of the cosmos, both in the sense of, you know, the structure of the cosmos, the shape of space, and the potential for — maybe there are more dimensions of space. Maybe space and time are emergent from some more abstract phenomenon. Maybe we’re gonna figure that out through things like holography and black holes. And there’s a whole other field that we can go into that I don’t want to get too big into right now. You know, so maybe we’ll learn something about the fundamental structures of reality. Maybe we’ll learn what dark energy is. Maybe we’ll learn what dark matter is. Maybe those things will inform our understanding of fundamental particle physics. Maybe we will get more information about the very, very early universe, and we’ll learn something about how the initial conditions for our universe were set up.
(41:45) All of those are super exciting in their own way, right? Every piece of that is something that would be tremendously important for physics, that would revolutionize how we think about the universe in really important ways. And as a side effect, we would learn a little bit about how our universe might end, what our ultimate fate might be. So I think there are very few people who are, you know, really, their main focus is what’s going to happen to the universe? How are we going to end? Really, it’s these other questions that get to the fundamental nature of reality, the evolution of the cosmos, the origins of the cosmos. And those all feed into these big questions about where are we going? What’s gonna happen next?
Strogatz (42:27): Wonderful. Well, we’ve been talking with theoretical cosmologist Katie Mack, author of the book The End of Everything (Astrophysically Speaking). Thanks so much for joining us today. Katie,
Mack (42:38): Thanks for having me. This was a really fun conversation.
Announcer (42:40)
Quanta Magazine is an editorially independent online publication supported by the Simons Foundation to enhance public understanding of science.
Strogatz (42:57): The Joy of Why is a podcast from Quanta Magazine, an editorially independent publication supported by the Simons Foundation. Funding decisions by the Simons Foundation have no influence on the selection of topics, guests, or other editorial decisions in this podcast, or in Quanta Magazine. The Joy of Why is produced by Susan Valot and Polly Stryker. Our editors are John Rennie and Thomas Lin, with support by Matt Carlstrom, Annie Melchor and Allison Parshall. Our theme music was composed by Richie Johnson. Special thanks to Bert Odom-Reed at the Cornell broadcast studios. Our logo is by Jaki King. I’m your host, Steve Strogatz. If you have any questions or comments for us, please email us at [email protected]. Thanks for listening.