Moonlighting Genes Evolve for a Venomous Job
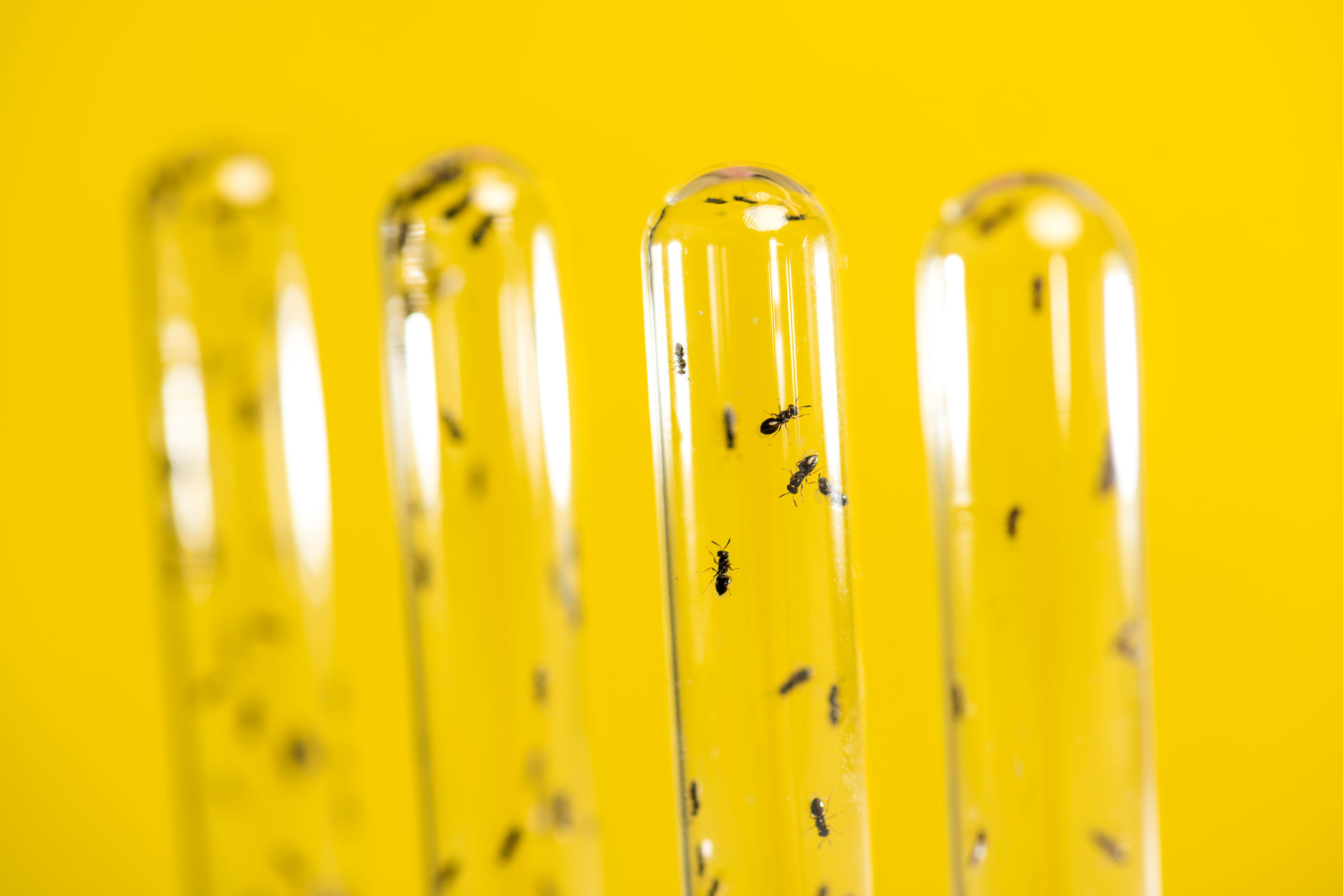
Tiny parasitoid wasps (Nasonia vitripennis) in a laboratory at the University of Rochester harbor a big secret. The genes that make their venom seem to have arisen by a novel mechanism that might prove to have wider evolutionary significance.
J. Adam Fenster / University of Rochester
Introduction
Venoms are among nature’s fiercest adaptations. The geographer’s cone snail, for example, only injects about a tenth of a milligram of venom when it stings, and yet, this is more than enough to kill a person in under an hour. These chemical cocktails contain some of the most potent compounds known, and their fearsome power has awed people since the dawn of history. It wasn’t until modern advances in genetics, though, that scientists were able to study how the genes encoding for such potent toxins arise, providing glimpses into the workings of evolution at the molecular level. From such studies came the current canonical model of how venom genes evolve through the chance replication and mutation of genes for enzymes, peptides and other proteins.
But new findings published today in Current Biology challenge this model, finding that the majority of toxin genes for parasitoid wasp species are instead “moonlighting” from other physiological roles. A further exciting implication is that if this discovery is relevant to compounds other than venoms, it might be a pathway that nature uses to develop other evolutionary solutions rapidly.
“I’ve been working on parasitoid wasps for a very long time,” remarked Jack Werren, a professor of biology at the University of Rochester. His fascination with these animals centers on their specialized venoms, which allow the wasps to be masterful physiological puppeteers. Parasitoid wasps are an enormous group of between 100,000 and 600,000 species that are parasitic when they are larvae, living on or frequently inside a host they eat alive. As free-living adults, they must find and subdue an appropriate creature to play host to their young, which they do with the aid of behavior-altering venoms. The emerald cockroach wasp, for example, transforms its formidable targets — cockroaches many times its size — into complacent meals for the wasps’ hungry offspring by manipulating the animals’ brain chemistry. The Glyptapanteles wasp goes even further, turning its caterpillar offerings into zombie bodyguards that protect the young wasps that have just eaten their way out of the caterpillars’ tissues. Another wasp, Reclinervellus nielseni, forces its arachnid victims to transform their webs into sturdy nests that will continue to protect the wasp larvae after the spiders expire.
“The venoms of parasitoids are quite different from those of most of the venomous animals that have been studied because they’ve evolved to manipulate metabolism” rather than to kill outright, Werren explained. He and two postdoctoral fellows in his lab, Ellen O. Martinson and Mrinalini (now at the National University of Singapore), were interested in understanding the diversity of toxins in parasitoid venoms and how those toxins evolve. They and their colleagues started by assembling genomes for several closely related wasp species, and they found something striking: Even close relatives among the wasps shared only about 30 to 40 percent of their venom genes. That surprisingly low number suggested the evolution of new species was accompanied by rapid turnover of the venom genes, with old genes being abandoned and new ones with novel venom functions suddenly arising. “Our next question was, okay, well what happened?” Werren said. “These genes that are being picked up, where are they coming from? And that got us into this broad question of: How do new genes’ functions evolve?”
Based largely on studies of snakes, spiders and other species dangerous to our own, it is thought that most venom genes arise through the mechanism of gene duplication followed by mutation and repurposing (which scientists refer to as neofunctionalization). The process begins when a gene for a molecule with a potentially toxic function, like a protein-chopping enzyme, is accidentally duplicated, typically during the formation of egg cells and sperm. The extra copy, free of the burden of performing the original gene’s biological duties, can accumulate changes through random mutations. Those changes may render the duplicate gene or its protein worthless, and it may disappear. Sometimes, however, those changes alter the protein in such a way that it becomes a useful toxin — and voilà, a venom toxin is born.
But when Martinson, Mrinalini, Werren and their colleagues compared the venom proteins and genes from four closely related species of parasitoid wasps, that’s not what they saw. In stark contrast to studies of other venomous animals, they found that nearly half of the 53 most recently recruited venom genes uncovered through their genetic analyses were single-copy, meaning they were not duplicates of other genes with which evolution had tinkered. In fact, less than 10 percent of the toxin genes clearly arose through duplication and mutation.
The team then wanted to understand how these single-copy genes went from performing ordinary, nonvenom functions to acting as toxins. And again, the results were surprising. “When we first started doing this, we were actually looking at this all wrong,” Werren said. “We thought that what we were seeing was the rapid specialization of genes as venoms, and loss of their other function.” But when they looked at which tissues in the wasps’ bodies were expressing the genes, “we discovered it wasn’t that.”
Instead, Werren likened the functionality of these single-copy genes to “moonlighting” for extra cash, with the genes taking on a “night job” in the venom gland in addition to their “day job” elsewhere in the body. The genes were routinely expressed to some degree in various tissues during stages of larval or adult development. The venom glands simply expressed the genes much more abundantly and steadily. Consequently, the gene’s protein — which had a benign physiological function elsewhere in the wasp body — reached a concentration with toxic properties in the venom. “That’s why a lot of this is expression evolution,” Werren explained. “The protein isn’t changing much. It’s just its expression pattern that’s changing to make it a venom.”
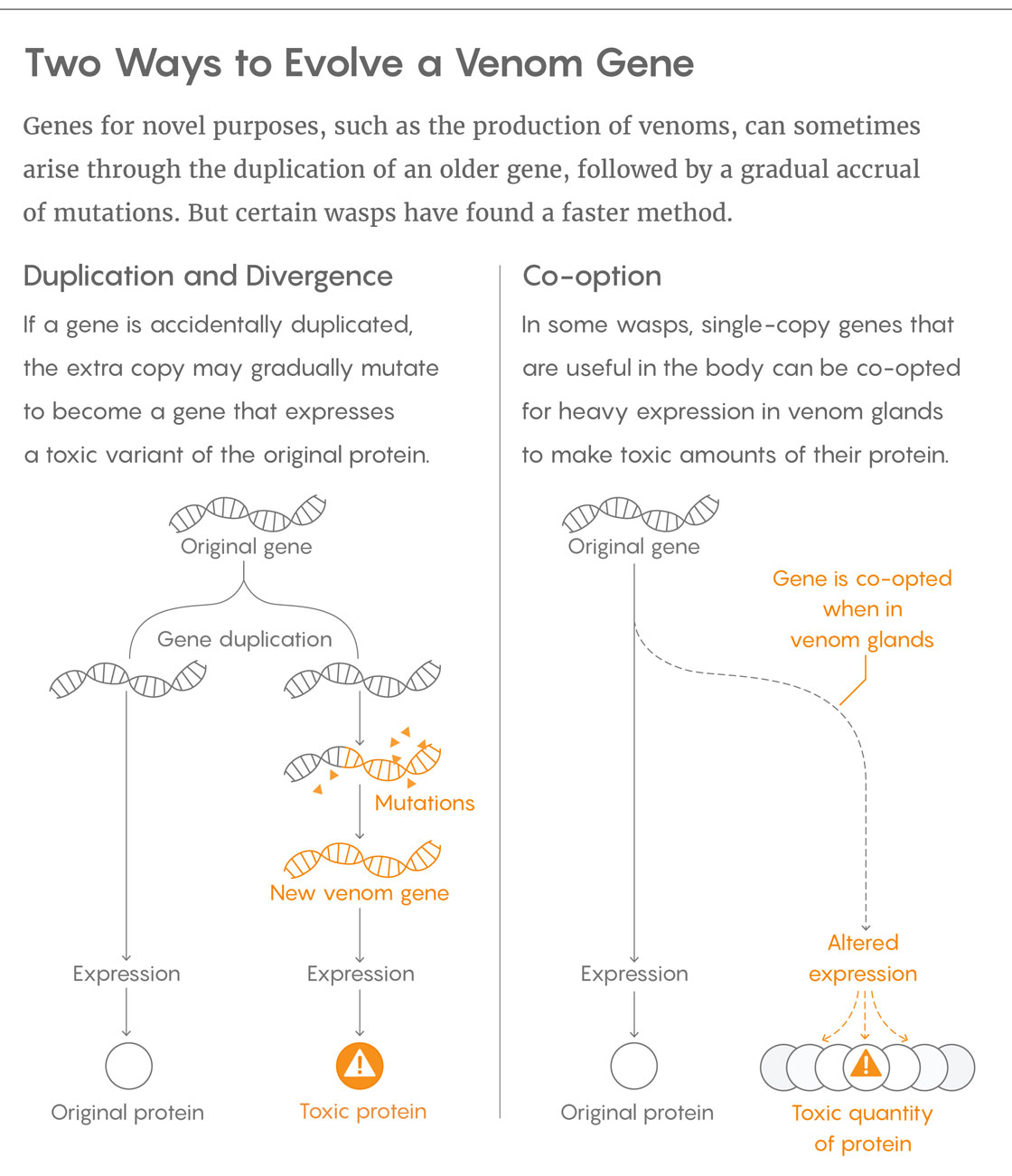
Lucy Reading-Ikkanda/Quanta Magazine
The lack of change is also very different from what is expected of venom genes. Implicit in the duplicate-and-mutate model is the assumption that the toxins need to evolve rapidly to be effective because many venomous animals can be locked in an arms race with their toxins’ targets. If the venoms don’t evolve quickly enough, then the predators or prey on which they’re intended to act will evolve a countermeasure and render the toxic advantage moot. And to date, biologists studying other species have seen venom genes evolve at a breakneck pace: The conotoxins employed by cone snails, for example, are known to mutate rapidly.
But the findings suggest that the wasps don’t need mutations in the venom toxin genes to switch from one host to another, or to keep pace with their current hosts. They just need to be able to co-opt and drop genes for use in making venoms quickly.
The results open the possibility that scientists have been overlooking a major pathway for the origins of toxin genes. “These findings raise important questions relating to the processes that other venomous animals may have used to generate their venom toxins,” wrote Nicholas Casewell, senior lecturer and Wellcome Trust research fellow with the Alistair Reid Venom Research Unit at the Liverpool School of Tropical Medicine, in a summary dispatch published alongside the Current Biology paper. “While gene duplication and alternative splicing are typically invoked as major mechanisms underpinning protein neofunctionalization, this study suggests that the process of co-option should be re-evaluated as a potentially important method by which genes can acquire novel functions.”
Bryan Fry, head of the Venom Evolution Laboratory at the University of Queensland, generally agreed with that view in comments emailed to Quanta. The work by Werren and his team is a “very intriguing paper that reinforces that the more we learn about venom evolution, the more we realise how little we know. Parasitoid wasps were already known to have very weird strategies and this paper reinforces just how uniquely divergent they are.” Whether co-option occurs more widely needs to be determined, he wrote.
“It had not particularly occurred to me as a likely mechanism of venom evolution,” admitted Wolfgang Wüster, a lecturer at Bangor University. He also isn’t convinced that this mechanism of gene evolution is prominent in other venomous groups. “I suspect it will remain unusual,” he said. “As the authors point out, parasitoid venoms act in a much subtler, more fine-tuned manner than the better known predatory or defensive venoms of things like snakes, spiders or cone shells.” That unique ecological role for the wasps’ venom could explain why these animals rely so heavily on a different mechanism for gene evolution than other venomous species. “Extended anaesthesia is much more difficult and requires much more finesse than killing something — ask any surgeon,” Wüster said.
Like Wüster, Werren considers the idea that co-option is rare among venom genes to be “one hypothesis that would have to be explored.” Nevertheless, he thinks it’s likely that this mode of evolution could have been underestimated because other studies have rarely compared evolutionary next of kin. “If you look at species that are very, very divergent from each other, you might find a certain subset of genes are shared and others [are] not, but you don’t really get the fine-scale picture.
“It may be that this process isn’t as important in snakes,” he said, but he noted that “a lot more work has to be done on looking at really closely related snake species before we can say for sure.”
Werren believes that the role of gene moonlighting might not stop with venoms. Gene moonlighting can occur merely through changes in expression, which may result from as little as a single mutation; it does not require the meandering process of random alteration and selection implied by the duplication and neofunctionalization model. Co-option is therefore likely to be a much faster mechanism for adaptation. “For species that have a very rapidly changing environment, this process of co-option of single genes may be fairly important. It just hasn’t been looked at that much,” he remarked.
“Is this a general function or is that something that only occurs in these specialized organs?” he asked. “I’m looking forward to addressing that question in the next several years, but I think it’s going to be a fairly broad mechanism, particularly when organisms are subject to rapid selection.”
“This paper is another nice illustration of one of the joys of biology,” Wüster said. “Just when you think you have found a generalisation of how animals accomplish something, something else pops up that does it completely differently.”
Corrections: This article was revised on June 26, 2017, to acknowledge Mrinalini as a co-lead investigator on this project. Also,
the lower limit of the number of parasitoid wasp species, which originally appeared as “100,00,” was corrected to 100,000.
This article was reprinted on ScientificAmerican.com.