Protein Blobs Linked to Alzheimer’s Affect Aging in All Cells
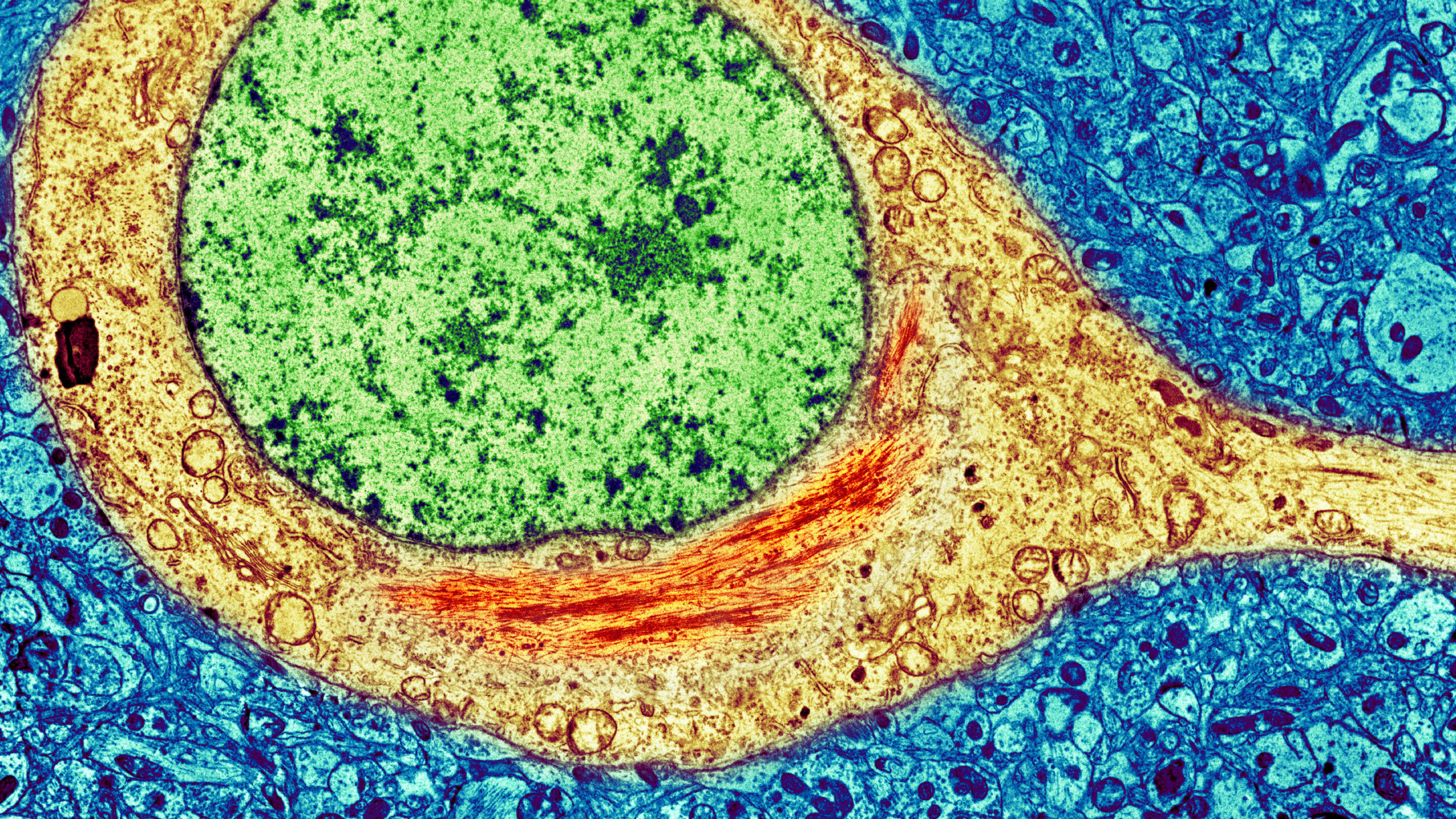
In this image of a neuron from the brain of an Alzheimer’s disease patient, abnormal aggregates of the protein tau appear as stacks of red fibers near the cell’s nucleus. Such aggregates are hallmarks of the disease, but protein aggregation seems to occur with aging throughout the body.
Thomas Deerinck, NCMIR / Science Source
Introduction
The aging brains of people with Alzheimer’s, Parkinson’s and other neurodegenerative diseases are suffused with telltale aggregates of proteins in or around their neurons. How these protein clumps might be harming the neurons is often still unclear, but they are hallmarks of the conditions — and until now, they have been associated almost exclusively with elderly brains.
But a recent study by a team of Stanford University researchers suggests that protein aggregation may be a universal phenomenon in aging cells and could be involved in many more diseases of aging than was suspected. Their discovery points to a new way of thinking about what goes wrong in cells as they age and, potentially, to new ways of staving off some consequences of the aging process.
“This is widespread — it’s not just one specific tissue, it’s lots of different tissues,” said Della David, a researcher on aging at the Babraham Institute in Cambridge, England, who was not part of the study.
The research also highlights that protein aggregation is tightly bound up with essential mechanisms that allow cells to regulate their physiologies with exquisite delicacy. Biologists will need to assess carefully, possibly on a case-by-case basis, whether protein aggregates represent a threat to cells or a defense they have created.
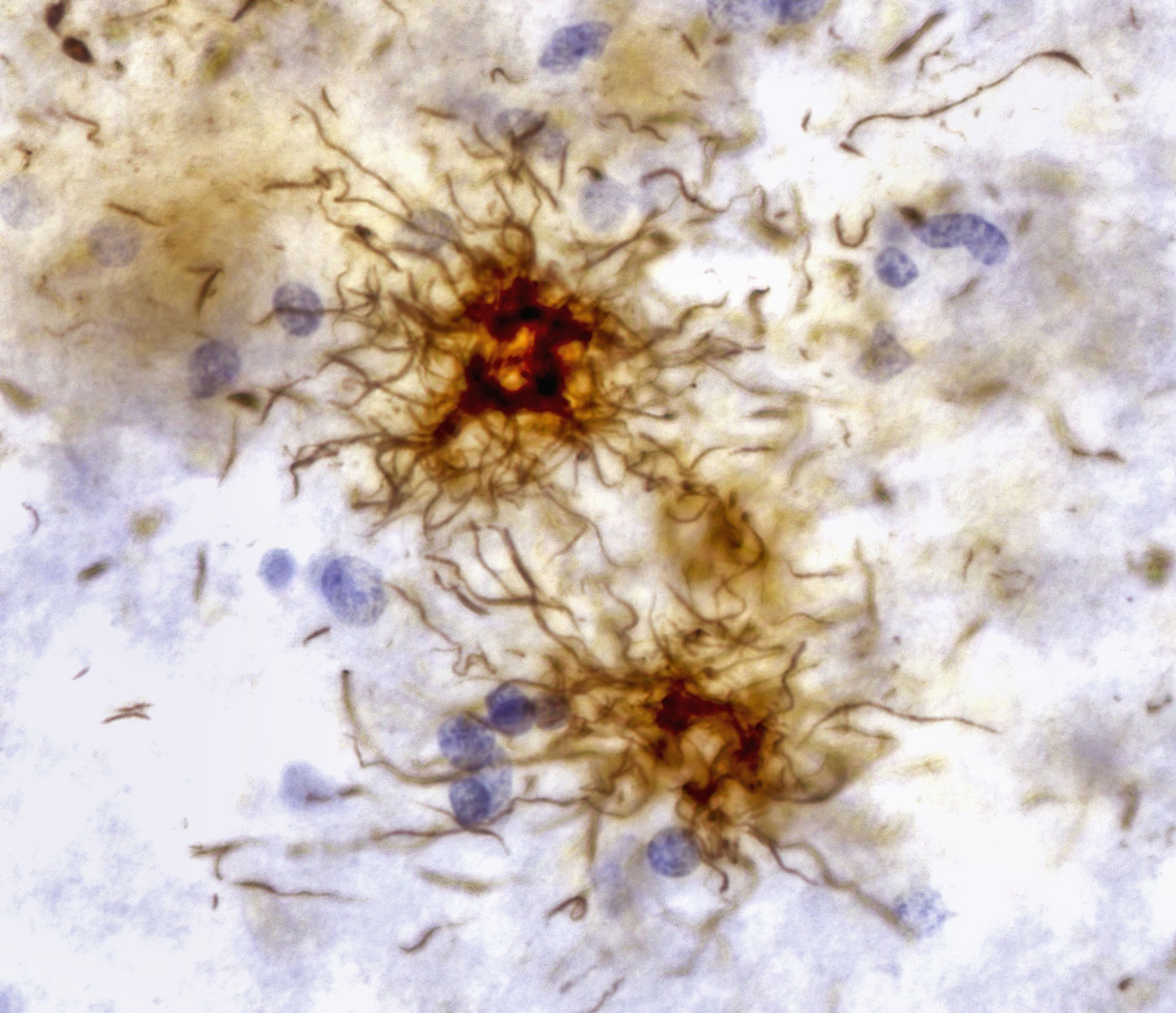
In this section of brain tissue from a patient with the neurodegenerative condition called progressive supranuclear palsy, aggregates of tau protein (brown) choke the spaces between neurons.
Alvin Telser / Science Source
The new work, which was posted to the biorxiv.org preprint server in March, is the first attempt to quantify how much protein aggregation occurs throughout the body during the natural aging of a vertebrate animal — in this case, a very short-lived fish. The study showed that protein aggregation probably contributes to the gradual deterioration of many tissues over time. The findings even offer a hint about why these aggregates are so much more obvious in the brain than in other tissues: It may be because brains have been evolving so rapidly.
Dan Jarosz, the Stanford systems biologist who oversaw the experiments with his geneticist colleague Anne Brunet, wasn’t prepared for how many proteins aggregated in the aging fish — or for how often those same proteins, in mutated forms, are linked with degenerative diseases. “It has made me wonder whether many more diseases of age that we don’t presently connect to protein aggregation might, in fact, involve it,” he said.
Clues from a Fish
The African turquoise killifish lives in transitory ponds in East Africa that form during the rainy season. As the fish nears the end of its 4- to 6-month life, it develops a range of age-related diseases, including cataracts and brain-related changes that resemble neurodegenerative disorders like Alzheimer’s in humans. Its brief life span — much shorter than that of a lab mouse, for example — and rapid natural aging make it an ideal model for studying aging in vertebrates.
“What’s striking about this fish is that it’s not just protein aggregation or heart failure or brain dysfunction that occur with aging,” said Dario Valenzano, an evolutionary biologist at the Max Planck Institute for the Biology of Aging and the Leibniz Institute on Aging, both in Germany, who did his postdoctoral training with Brunet. “Pretty much any organ and tissue that we look at will undergo some quite catastrophic transformation during aging.”
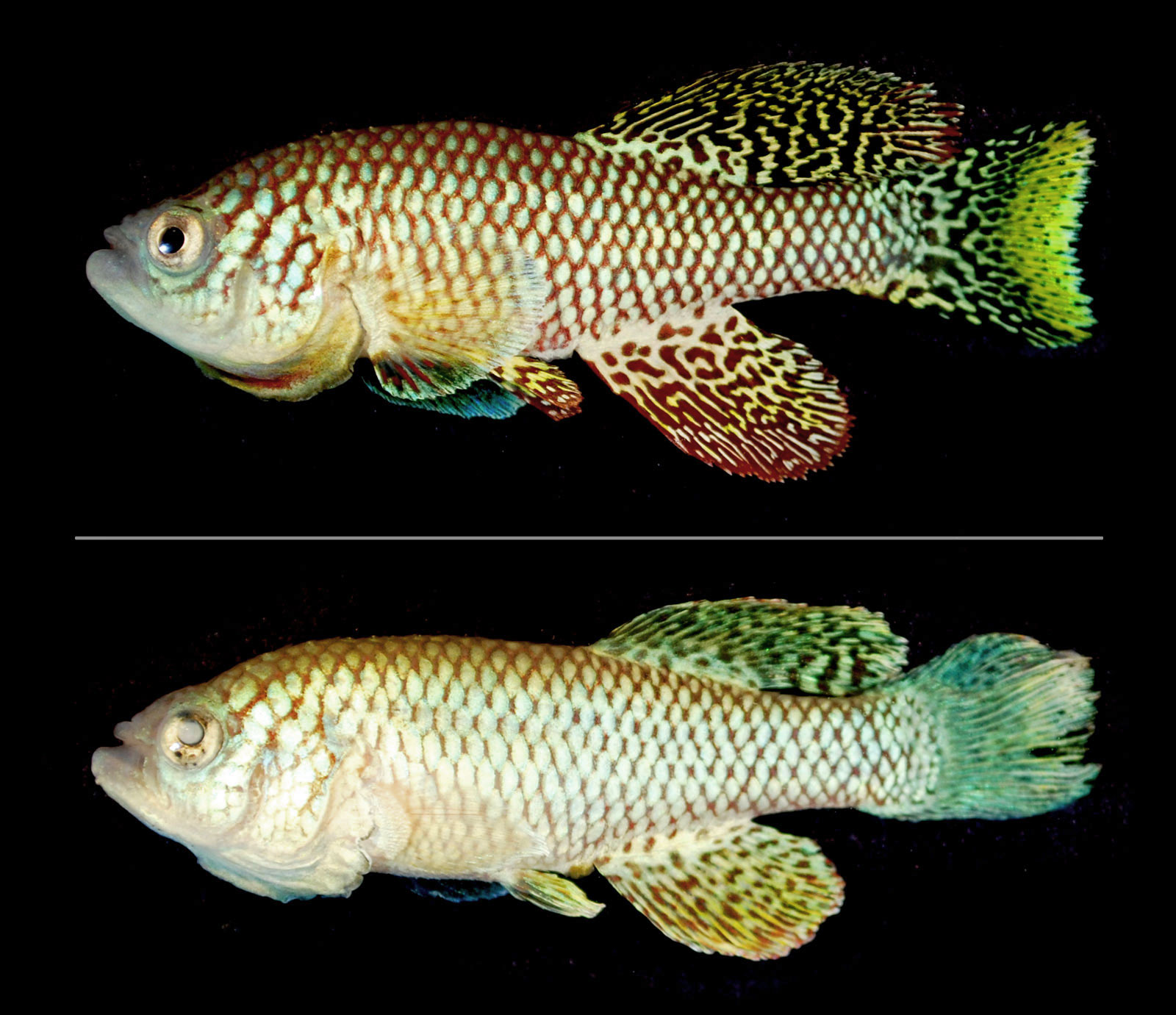
The African turquoise killifish is a vertebrate with an exceedingly short lifespan of just 4- to 6-months. As it passes from youth (top) to senescence (bottom), it develops many health problems associated with old age in humans.
(from top) MDI Biological Laboratory; Itamar Harel
The Stanford team conducted an extensive analysis of the proteins in killifish at various stages of youth and maturity. In the aging killifish, they discovered protein aggregates in all the tissues that they looked at: not only the brain but also the heart, gut, liver, muscle, skin and testis. More than half of the aggregating proteins seemed to show an intrinsic tendency to aggregate in further experiments.
But precisely which proteins aggregated differed substantially from one tissue to another. Many of the proteins were expressed at essentially equivalent levels in multiple tissues, yet while they aggregated in one they did not clump at all in others.
“The extent of tissue specificity of the aggregating proteome is amazing,” David said. The reasons for those differences, she and other researchers think, reflect how cells maintain the quality of their proteins. Cells have elaborate machinery for ensuring that the long, chainlike peptide molecules making up proteins get folded properly, and even for making sure that the peptides are eventually chopped up for recycling. But tissues may vary in how much they rely on various aspects of the protein quality control process, and those emphases may change with age, Jarosz said.
“That is really important, because a huge mystery in human biology is why these neurodegenerative diseases are so tissue specific,” said Cynthia Kenyon, vice president of aging research at the biotechnology company Calico Life Sciences, who was not involved in the Stanford paper. No one really knows, for example, why the amyloid protein plaques of Alzheimer’s disease form in the hippocampus of the brain and the aggregates in Parkinson’s disease are specific to dopamine neurons. The possibility that various cells maintain their protein quality differently “at least provides a possible explanation for why different tissues should behave so differently,” she said.
The Importance of Quality Control
There’s good evidence from studies of worms and flies that if the machinery that preserves the stability of proteins is perturbed, animals age more rapidly. If the protein quality-control pathways are genetically enhanced, the animals tend to live longer. None of this means that protein aggregation causes aging, but it strongly implies that the two are tightly correlated.
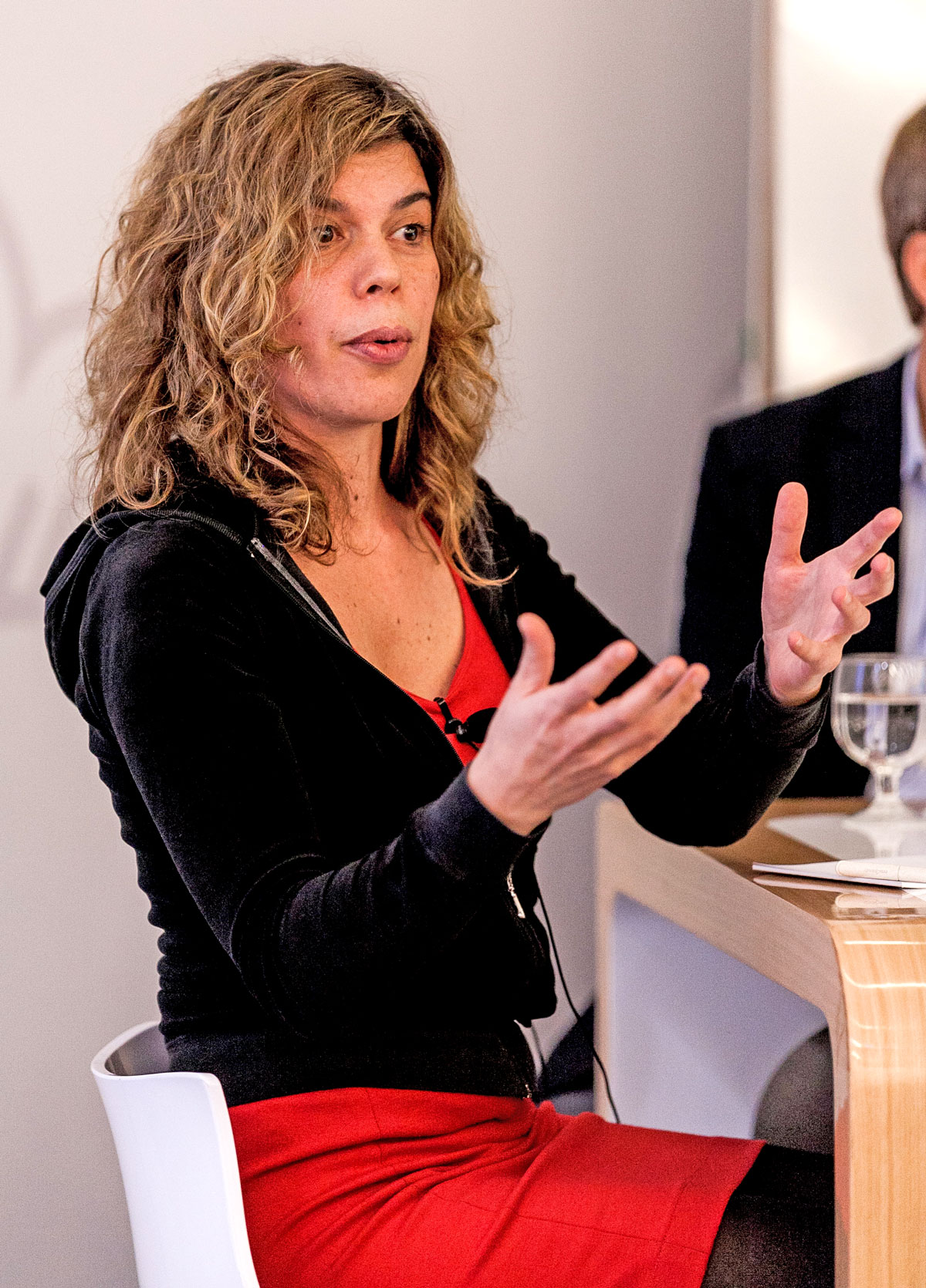
Anne Brunet, a geneticist at Stanford University, was one of the co-leaders of the project that quantified protein aggregation throughout the body of a vertebrate — the African turquoise killifish — for the first time.
Jakob Polacsek, World Economic Forum
To further probe the relationship between protein aggregation and aging, the Stanford researchers looked more closely at the proteins in a mutant variety of killifish that ages unusually quickly. These fish have a mutation in their gene for the enzyme telomerase, which preserves the length of dividing chromosomes; animals with telomerase mutations typically age quickly.
Jarosz said that he and his colleagues expected to find that in the gut and other tissues that grew or replaced themselves rapidly, there would be fewer aggregates: Extra cell divisions would give rapidly growing tissues more opportunities to clear away aggregates and reset themselves. But the opposite was true: Fast-growing tissues had more misfolded and aggregated proteins, and they aged more rapidly than tissues that grew slowly.
Once again, problems with the cell’s control over the quality of its proteins may be the explanation. If cells lose control over the processes that maintain the quality of their proteins, more damage from aggregates may build up with each cell division. Tissues that grow rapidly may age faster because they have more chances to accumulate that harm.
Condensation, Aggregation and Prions
Why proteins sometimes aggregate is complicated. Surprisingly, part of the answer turns out to be deeply connected to an essential mechanism called condensation that cells use to control their proteins.
The complex 3-D shapes that peptides fold into were historically seen as dictating the activities and functions of the proteins they made up. But in the last decade or so, it was discovered that a growing list of proteins have an “intrinsically disordered” region that doesn’t fold into a stable shape. Under the right conditions, multitudes of these proteins gather into droplets, or condensates — a reversible process akin to the “phase separation” that makes oil form droplets in water. It can enhance enzyme activity by concentrating enzymes together with their substrates or suppress activity by sequestering enzymes away from their substrates. By altering the local concentration of substrates and enzymes within themselves, cells can use condensates to finely tune their protein activity.
But the disordered regions of proteins can also cause them to stick together more permanently as aggregates, gumming up cells and wreaking havoc. Worse, some defective proteins not only misfold and aggregate themselves but also cause other proteins of the same type to misfold, leading to a chain reaction of aggregation. This is conceptually similar to what happens in “mad cow disease” and variant Creutzfeldt-Jakob syndrome, in which abnormally folded proteins called prions catalyze a wave of abnormal protein aggregation in the brain.
Condensation is therefore a control mechanism that comes with risks. But in evolutionary terms, its advantages are apparently so substantial that the cost — a vulnerability to many aging-associated diseases — seems to be worth paying, Jarosz said.
A clear illustration of this emerged in a second preprint posted in March, in which the Stanford team homed in on a protein called DDX5 that aggregates in aging killifish brains. DDX5, which is most active in its condensate state, serves a variety of important functions in the body, often helping to make sure that other proteins are made properly. From its amino acid sequence, the researchers predicted that DDX5 was likely to behave like a prion, and their subsequent work confirmed that it does: One misfolded DDX5 protein promotes the misfolding and aggregation of other DDX5 molecules.
But the aggregation doesn’t stop there: The Stanford researchers found a variety of other proteins in the clumps of DDX5 too. Aggregates can sometimes act as “sticky blobs” that trap other proteins, indiscriminately interfering with cellular functions, explained John Labbadia, whose laboratory at University College London studies protein quality control and aging.
“It suggests that we have these … proteins that aggregate with age and that can actually catalyze further aggregation of proteins in a prionlike manner, which was not shown before,” he said.
Merrill Sherman/Quanta Magazine
The Stanford team carefully established which region of the DDX5 protein makes it possible for condensation to control its activity — and it turned out to be the same region that also makes it prone to aggregation. Control over the protein’s natural function and its tendency to aggregate are inextricably linked. “It’s a Catch-22,” Labbadia said.
“One of the fascinating shifts in mindset for me is that the disordered domain isn’t required for activity as very narrowly defined,” Jarosz said. “But in terms of how that activity is really deployed in the living system, it’s actually extremely important.”
Pathological or Protective?
Exactly what triggers aggregates to form, and how much trouble they cause for cells, remains “a huge, fantastic, big controversy in the field,” said Kenyon. On the one hand, aggregates sequester DDX5 and other proteins, effectively eliminating important cellular functions. But aggregates may also have a protective effect on cell survival.
A good example of the protective effect emerged from studies of the huntingtin protein, which is most abundant in the brain. Huntingtin is essential for the healthy development of nervous systems, but in people with Huntington’s disease, a mutation causes the huntingtin protein to be abnormally long. The long protein then gets chopped up into smaller, toxic segments that damage the nervous system.
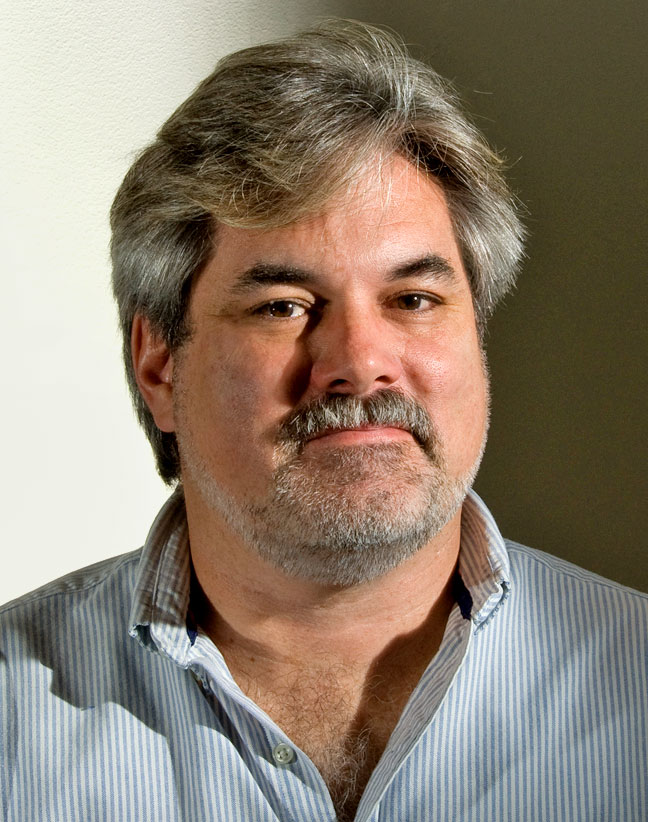
Steve Finkbeiner, a researcher at the Gladstone Institutes and the University of California, San Francisco, showed that the protein aggregates that form in neurons in Huntington’s disease may have a protective influence on the cells.
Chris Goodfellow/Gladstone Institutes
In 2004, Steve Finkbeiner, a researcher on aging at the Gladstone Institutes and the University of California, San Francisco, was studying aggregation of huntingtin protein in cultured neurons. His team showed that although all the neurons expressing the abnormal huntingtin protein died over time, the neurons that had aggregates of huntingtin survived longer than those that did not.
“It was the first evidence that [aggregate] formation was a coping response to other submicroscopic forms of the misfolded protein that were causing the trouble,” Finkbeiner explained in an email to Quanta.
He and others have shown since then that this protective aggregation response occurs in other neurodegenerative diseases as well. It may explain the repeated failure of experimental trials for treating Alzheimer’s disease by targeting plaques, he said: If the amyloid plaques characteristic of the disease form to protectively bind up the defective protein, then breaking up the plaques might do more harm than good.
“It is a hard concept for humans to grasp, since it seems intuitive that things that look abnormal should be ‘bad’ and pathogenic,” Finkbeiner wrote. “But biology is complex, full of many feedback loops, so it is important that people don’t get fooled jumping to conclusions.”
A Universal Challenge With Many Solutions
The picture emerging clearly now is that protein aggregation isn’t a phenomenon restricted to neurodegenerative diseases: It is part of every cell that lives long enough to age. Many normal, developmentally important proteins like DDX5 have a tendency to aggregate, and coping with this clumping is a universal challenge that every cell has to address.
Since cells have been dealing with this problem for a very long time, preventing aggregation may have been a major force in the evolution of protein sequences. Because abundant proteins are prone to aggregation, and mutations increase that tendency, natural selection against mutations in abundant proteins is likely to be very strong. (That conclusion is supported by the observation that in young animals, more abundant proteins tend to have lower mutation rates.) So scarce proteins may evolve more quickly than abundant proteins, and a faster evolutionary rate should correlate with a propensity to aggregate.
Brunet and Jarosz observed that this effect was most pronounced in the brain of the killifish. The researchers speculated that those aggregating proteins may have been keys to innovations in the organ. If so, the evolutionary changes in the brain that made it such an important organ in vertebrates may also have made the organ more vulnerable to degenerative diseases caused by aggregation.
Indeed, it’s likely that every tissue and organ has to find a different balance or trade-off between doing its job and managing protein aggregation, Jarosz said. Every tissue has unique functional requirements and constraints to obey: Intestinal cells turn over constantly; endocrine cells make and secrete hormones; immune cells spring to action when they detect invaders; the brain processes information. Different jobs demand different proteins, which means the evolved strategies for coping with protein aggregation will vary from tissue to tissue and from animal to animal. Because the vertebrate brain has in the relatively recent past evolved so much more extensively and quickly than, say, the muscles, its protein quality control machinery may not yet have had enough time to evolve adequate protections against the aggregation of relatively new proteins.
Still, the fundamental problem of protein aggregation is there for all organisms every day, not just during interludes of disease or massive stress. The prionlike DDX5 and similar proteins “have an intrinsic propensity to aggregate, and the organism strives to protect itself against the aggregation,” David said. “It’s something physiological that we all have to deal with.”
And the fact that protein aggregation throughout the body is a factor in the aging of organisms as disparate as yeast, worms, flies, fish, mice and humans, she added, “means that we, as a field, should be paying a lot more attention to this.”