Soil’s Microbial Market Shows the Ruthless Side of Forests
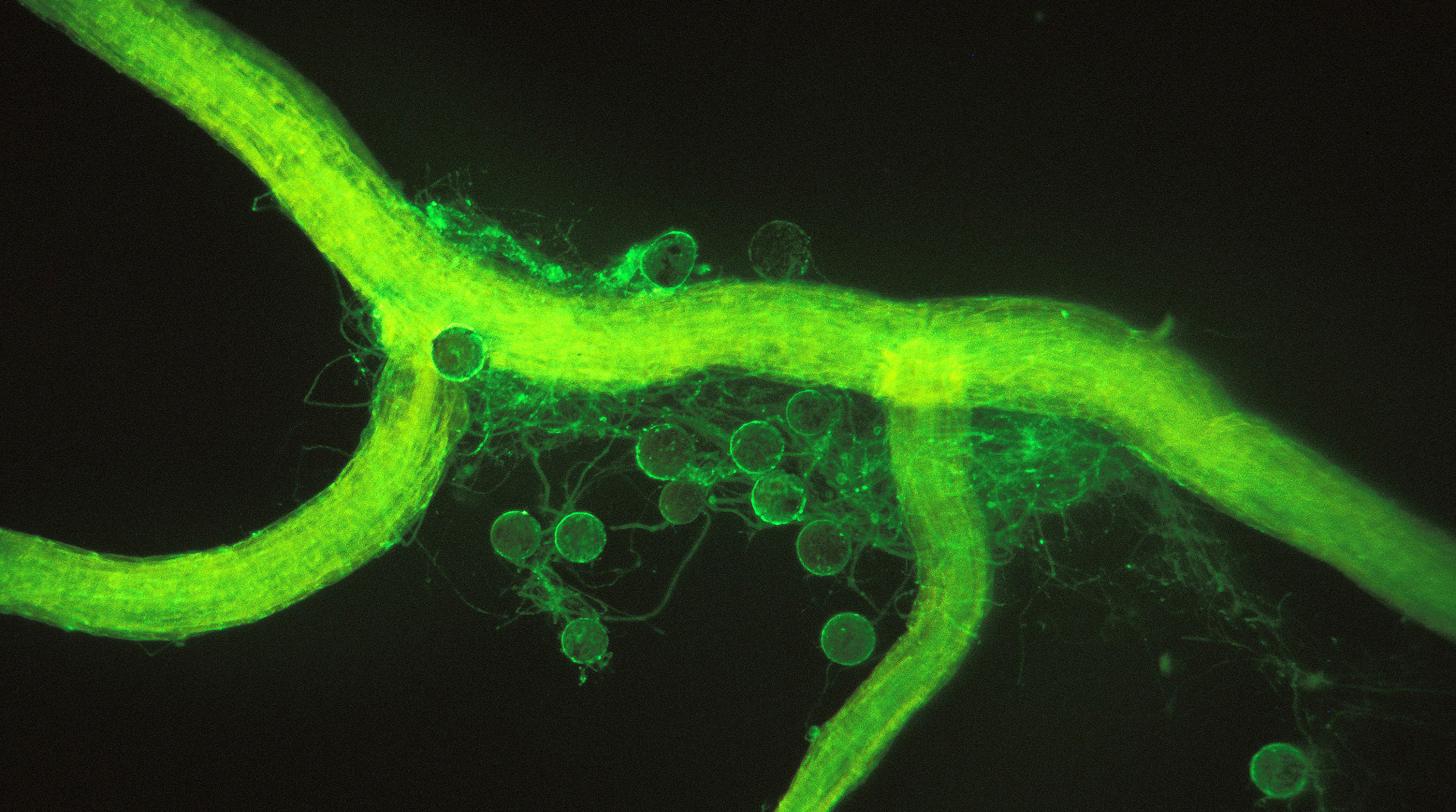
Round spores and threadlike filaments from a mycorrhizal fungus grow around this plant’s root. The complex subterranean interactions between symbiotic fungi and plants can be both cooperative and competitive.
Sara Wright/U.S. Department of Agriculture
Introduction
Beneath the green vegetable world we see is a dark microbial world we don’t. The crops we eat, the forests that sustain us and most other life forms, even the regulation of Earth’s climate — all benefit from a shadowy network of fungi and bacteria that mobilize soil nutrients and trade them with plants for sugars and fats. And yet the workings of this subterranean society are almost unknown to scientists. For example, researchers just mapped for the first time the global distribution of three major groups of these microbes. Even in 2019, what lies beneath our feet remains a true scientific frontier.
Despite this epistemological murkiness, public interest in the underground ecosystem has exploded. TED talks and bestselling books extol the benevolent, cooperative “wood wide web” of subsurface organisms that communicate, share nutrients and sustain each other.
Toby Kiers, an evolutionary biologist at VU University Amsterdam, is at the vanguard of a new generation of scientists questioning that gauzy view. Through innovative and groundbreaking studies, Kiers and her collaborators have gathered evidence that plants and their fungal conspirators are not just cooperating with each other but also engaging in a raucous and often cutthroat marketplace ruled by supply and demand, where everyone is out to get the best deal for themselves and their kind.
Key to this picture is the revelation that the unseen underground world is just as complex, sophisticated and purposeful as the visible aboveground world we inhabit. Microbes are not simple, passive accessories to plants, but dynamic, powerful actors in their own right. Fungi can hoard nutrients, they can reward plants that are generous with their carbon reserves and punish ones that are stingy, and they can deftly move and trade resources to get the best “deal” for themselves in exchange.
Those are probably just the beginning of their talents. In a paper published in June, Kiers and her colleagues pioneered a method to illuminate the fungal marketplace in action — to make the invisible visible. The tantalizing research hints at a capability that has been suspected but never proved: that fungi might not be just nutrient traders but also sophisticated information processors.
Kiers is the first to admit that scientists have far to go in puzzling out the hidden rules of the tiny networked organisms that somehow support all the rest of us. “Doesn’t it strike you as odd?” she said. “We know so much about other types of networks. This is undoubtedly the most important network for our ecosystems, but we just don’t know anything about it. … It’s radically under-studied.”
Ancient Partners
When plants crept onto land some 500 million years ago, microbes were waiting. Fungi and bacteria struck up relationships with their new neighbors. Plants, after all, could do something most microbes could not: harness solar energy to split apart atmospheric carbon dioxide and construct energy-rich sugars and fats from the pieces. The microbes, in turn, had mastered the art of freeing up the nutrients that plants needed from the soil — phosphorus especially, but also nitrogen; there is evidence that microbes help plants gain access to water as well. Some 80 percent or more of today’s land plants form partnerships with fungi; still other plants partner with bacteria. If the soil were somehow purged of its microbes, the plant and animal worlds would take a big hit. The views of the great naturalist E.O. Wilson notwithstanding, it’s microbes, not insects, that run the world.
And yet the soil microbiome is little known and even less appreciated. There are reasons for this: Soil is opaque and microbes are, well, microscopic. They’re also hard to study; many won’t grow in the lab, and the wispy fungal networks that pervade soil break easily when extracted. Most of all, though, microbes confound our understanding of life, which has been shaped by our experience of the aboveground world. Some fungi don’t have proper cells, for example: Their DNA-containing nuclei float through threadlike subterranean networks that can be kilometers long. It’s often hard to say what it means for fungi to have sex, or even to define what constitutes an individual.
As scientists began to appreciate the importance of microbe-plant partnerships, at least in outline, many came to see the natural world as a cooperative, even communitarian kind of place. In the 1970s, the microbiologist Lynn Margulis and the chemist James Lovelock developed the Gaia hypothesis, which posits that Earth’s biosphere is, in some sense, a unified self-regulating organism. The existence of mutually beneficial inter-species and even inter-kingdom relationships fit right into this picture.
In the mid-1990s, a young biologist named Suzanne Simard, now at the University of British Columbia, decided to test this concept in a forest. “Some people thought I was crazy,” Simard (who did not respond to multiple interview requests) said in a 2016 TED talk. For her doctoral project at Oregon State University, she took carbon dioxide with radioactive carbon isotopes and injected it into bags installed around pint-size birch trees growing near Douglas fir seedlings. After a little while, she ran a Geiger counter along the Douglas fir trees, and the device beeped like crazy. Moreover, she found that the radioactive carbon could also flow from the Douglas firs to the birches if she planted the bags near the firs. She had discovered that the trees shared carbon via underground networks. Her findings, published in Nature in 1997, lit a fire under scientists and the public alike.
In describing what she found, Simard has emphasized the cooperation she views as inherent in nature. “A forest is a cooperative system, and if it were all about competition, then it would be a much simpler place,” she said to Yale Environment 360 in 2016. “Why would a forest be so diverse? Why would it be so dynamic?” In her TED talk, Simard referred to forests as “supercooperators” and made the bold assertion that trees don’t just cooperate but communicate. She described birches and Douglas firs as engaging in a “lively two-way conversation” mediated by their underground collaborators. “I had found solid evidence of this massive belowground communications network,” she said, adding later in the talk, “Through back and forth conversations, they increase the resilience of the whole community.”
Not long after Simard bagged saplings in the misty Pacific Northwest, Kiers decamped from Bowdoin College in frigid Maine to the warm and humid Barro Colorado Island in Panama. She had become fascinated by the underground world, and, at her undergraduate mentor’s suggestion, she spent a year at the Smithsonian’s renowned tropical field station in the main channel of the Panama Canal, studying how soil fungi help tropical trees grow. She then finished up her bachelor’s degree and headed to the University of California, Davis. There she began studying one of the world’s most famous mutualisms, the one between plants in the legume family — which includes important crops like soybeans and alfalfa and trees like the locust — and rhizobial bacteria. These specialized microbes nestle into spherical white nodules on plants’ roots and become nutrient factories, converting inorganic nitrogen from the air into biologically useful forms. They then trade the nitrogen to plants for carbon-rich sugars.
It seems like a balanced, helpful exchange between friendly partners. But to Kiers, the benevolent, cooperation-focused view promoted by thinkers like Margulis and Simard was suspect. Kiers saw instead a world ordered by individual interests, where potential cheaters lurked everywhere and species needed complex strategies to keep their trading partners in line.
“I had this realization … that I’m less interested in cooperation and I’m actually much more interested in the tension,” Kiers said. “I think there’s an underappreciation of how tension drives innovation. Cooperation to me suggests a stasis.”
As she soon learned, the legume-bacteria interaction is not so simple. A single legume plant can host 10 or more strains of bacteria. To Kiers, this evoked a concept from the ecologist Garrett Hardin, whose famous 1968 essay in Science, “The Tragedy of the Commons,” argued that individuals pursuing their own interests can destroy a common environment or resource. The legume plant itself could be seen as a commons, and any given bacterial strain could hoard nitrogen while continuing to feast on the plant’s sugars. “Why should they fix nitrogen — what’s in it for them?” asked Ford Denison, Kiers’ adviser, now an adjunct professor who runs an ecology and evolution lab at the University of Minnesota.
Along with Stuart West, an evolutionary theorist at the University of Oxford whom Kiers had met in Panama, she and Denison modeled the legume-rhizobia interaction mathematically, showing that if bacteria could “cheat” their plant hosts, the relationship would fall apart as more and more strains defected. Kiers, Denison and their colleague Robert Rousseau then designed an experiment that would essentially force some of the bacteria to cheat.
Kiers surrounded some nodules on soybean plants with an almost nitrogen-free air supply, making the bacteria in those nodules useless to the plant. She found that the plant reacted by shutting off the supply of oxygen to those bacteria, drastically reducing their reproduction. It seemed the relationship between the bacteria and the soybeans, far from being a happy friendship, was an uneasy détente, with the plant imposing crippling sanctions on any bacterial partners that failed to earn their keep. The paper, which was published in Nature before Kiers even received her doctorate, made a huge splash. “It’s the most cited paper in my career,” Denison said.
Kiers then switched from bacteria to fungi. While bacteria might nestle into the roots of select groups of plants, fungi are without question the masters of the underground domain. Certain fungi spread through vast areas and commingle with just about every plant they encounter, even sending thready tendrils known as hyphae directly into plants’ roots. (The name for these fungi — “mycorrhizae” — literally fuses the Latin myco-, meaning “of fungi,” with the Greek rhiza, or “root.”) Indeed, the mycorrhizal world forms a sort of inversion of the vegetable one, with branching fungal networks extending downward, mirroring the branching stems and limbs of the plants reaching skyward.
But what really distinguishes the fungal world is its diversity and complexity. A spoonful of soil contains more microbial individuals than there are humans on Earth. “It’s the most species-dense habitat we have,” said Edith Hammer, a soil ecologist at Lund University in Sweden. A single plant might be swapping molecules with dozens of fungi — each of which might in turn be canoodling with an equal number of plants. It’s a promiscuous party down there.
Faced with such overwhelming complexity, scientists must simplify, just not too much (as Einstein is alleged to have said). Kiers and her colleagues did this with a petri dish divided into three equal-size compartments, like a Mercedes symbol. In one, they grew carrot roots deprived of leaves along with fungal species known to associate with carrots. The fungal hyphae, but not the plant roots, were able to grow into the other two compartments to look for nutrients. The researchers gave a special “heavy” form of carbon (the isotope carbon-14) to the carrots; they also made phosphorus available to the fungi that reached into one of the other two compartments. In this way, the scientists could track the movement of sugars and nutrients through the simplified ecosystem. After some time, they measured the fungi’s growth and found that the fungi with phosphorus to trade received much more carbon from the plants.
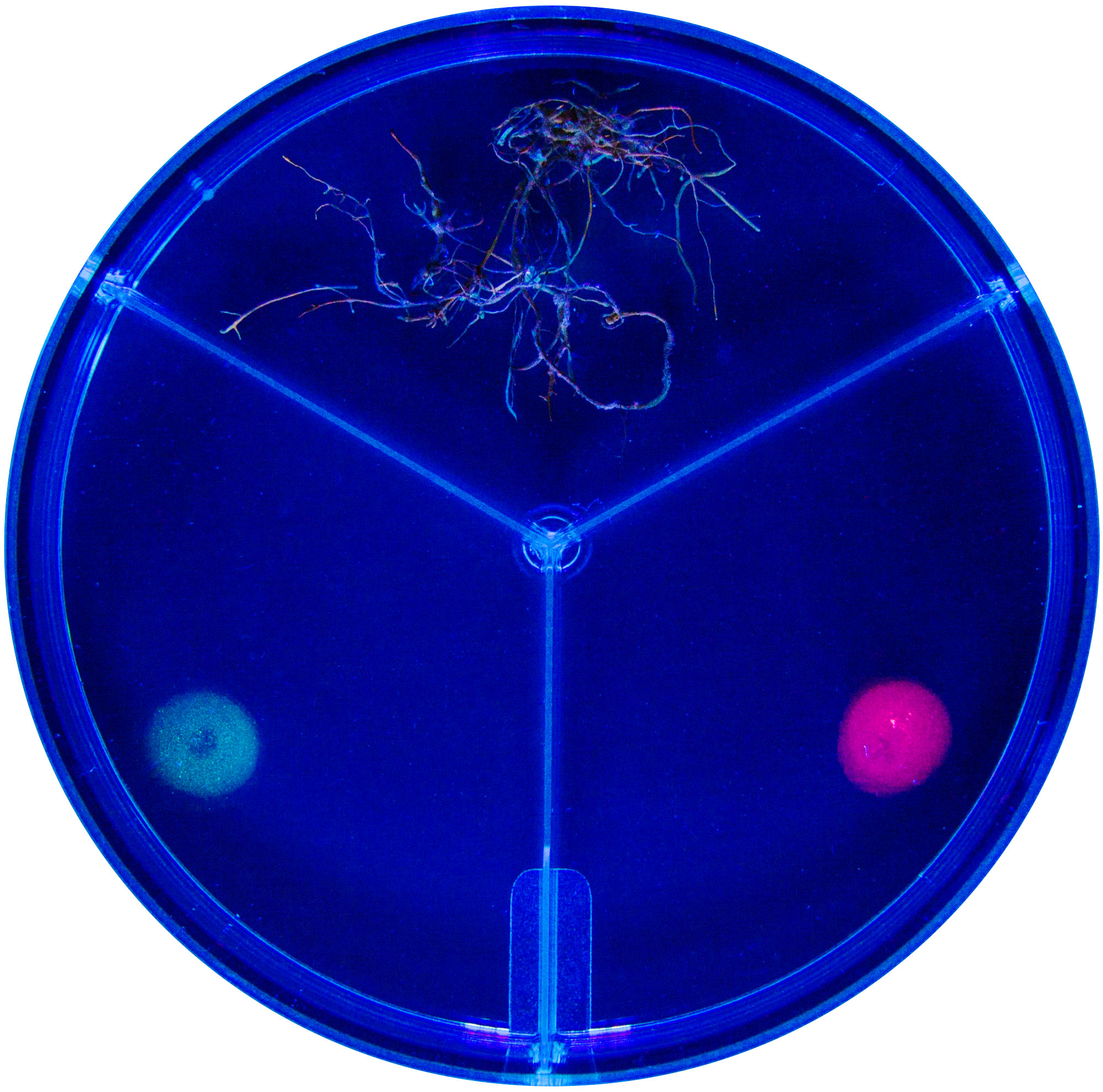
By growing carrot roots and fungi in segmented petri dishes that held unequally distributed nutrient resources, Kiers and her colleagues demonstrated that fungi can move resources to get higher payoffs from plants.
Courtesy of Toby Kiers
What Kiers’ team did next was the real coup. They inverted the setup: carrot roots with fungi in two compartments and one compartment that only the fungus could reach. They gave one carrot compartment more sugar than the other. They waited, and then measured. The plant with more sugar to trade had received far more fungal phosphorus (which in this experiment was recognizable as the “heavy” isotope phosphorus-32).
In 2011, Kiers’ team reported in Science that not only can plants reward high-performing fungal partners and punish poor performers, fungi apparently do the same.
Around the same time, Hammer reported evidence from experiments that fungi have a second trick: They can store nutrients when a plant isn’t paying well, withholding them until they get a better offer.
Together, the results turned scientists’ understanding of the plant-fungal relationship on its head. No longer could mycorrhizal fungi be seen as servants or passive accessories to their plant masters. Rather, life forms below the surface control their own fate, just as much as those above. It’s a dynamic marriage of equals.
“I don’t know if we should say that we enlightened the field, but I think a lot of people thought [fungi] were much simpler” and mostly only responded to plants’ signals, Hammer said.
The findings also established Kiers as an important thinker in her own right. “It’s pretty amazing that this one person provided the first solid evidence for sanctions or discrimination in what are arguably the two most important symbioses — the legume-rhizobia system and the mycorrhizae,” Denison said.
She followed up the lab experiment with a less artificial one: She grew plants connected to mycorrhizae in shade and in full sunlight. She found that the shaded plants, which photosynthesized less and thus had fewer sugars to share, received less phosphorus from their underground fungal counterparts.
She also began developing an economic framework for thinking about relationships between plants and fungi. Based on observations of the free-market system, Kiers suspects that what has stabilized plant-fungal mutualisms for at least 470 million years is not that individual organisms are committed to the good of the community, but rather that, in most cases, both plants and fungi benefit more from trading with each other than from keeping resources to themselves.
Economics provides “this huge body of literature we can borrow from that’s actually mathematical and predictable,” Kiers said. “It can be used as a tool to test some of these ideas.” For example, Kiers and her colleagues found that mutualisms sometimes break down when plants find another way to get the nutrients they need — by turning carnivorous and catching insects, for example. They published their findings last year in the Proceedings of the National Academy of Sciences.
Not everyone is convinced that the cutthroat economic world of markets and traders describes plants and microbes well. “I think it’s possible that sometimes the fitness interests of partners just happen to be really well aligned,” said Megan Frederickson, a professor of ecology and evolutionary biology at the University of Toronto who has argued in several papers that cheating is far less common in nature than Kiers believes. “I think some other people take the view that it’s probably impossible for two partners in any interaction to have perfectly aligned interests.”
That view has also come to dominate the popular literature. In 2016, the German forester Peter Wohlleben, drawing heavily on Simard and a few other scientists, published The Hidden Life of Trees. The book became an international bestseller. Wohlleben, a strong advocate for a communitarian view of nature, wholeheartedly promoted fungi’s supposed beneficence and cooperative nature, writing, “The fungi that populate [trees’ roots] seem to be intent on compromise.”
Kiers, characteristically, has turned to different sources for inspiration. A few years ago, she read the economist Thomas Piketty’s Capital in the Twenty-First Century, which emphasizes the role of resource inequality in shaping human societies. Kiers suspected that introducing inequality into her fungus-plant ecosystem would reveal novel insights. But she found herself facing the same challenge that had stymied so many scientists: She had no way to directly see what fungi were doing. She had gotten a lot of mileage out of measuring nutrients going in and seeing where they ended up, but what happened within the fungi themselves remained a black box.
“That’s how we start lab meeting every week: ‘If we can’t talk to them, how do we get at that question?’” Kiers said.
The breakthrough came unexpectedly. Kiers had gotten a Dutch government grant to work with an artist on a stop-motion video depicting phosphorus and other nutrients moving through the fungal network, using LEDs to represent nutrients. She showed the animation at a 2014 scientific conference. “Wouldn’t it be cool if we could do that?” she asked her audience.
Matthew Whiteside, a chemist then working at the University of British Columbia, approached Kiers afterward. “We really can do that,” he said.
Whiteside — who, incidentally, had known Margulis as a family friend and spoken with her about pursuing science — had developed a way to tag biomolecules with quantum dots, nanometer-scale bits of semiconductor that absorb certain short wavelengths of light (usually ultraviolet) and re-emit light, or “fluoresce,” at longer wavelengths. Kiers hired him. The two spent several years developing the technique and ensuring that they could distinguish the dots’ emissions from the plant cells’ natural fluorescence. They also had to ensure that the dots wouldn’t poison fungi or plants.
Kiers and Whiteside then set up another experiment in the three-compartment petri dish. They grew carrot roots with fungi in one compartment and allowed the fungi to expand into the other two. Then they introduced apatite, a phosphorus-containing mineral, into the fungi-only compartments. In one, they labeled the apatite with a red-fluorescing quantum dot; in the other they used a blue dot. They used specialized microscopes to quantify the emitted light.
At first they gave each fungal compartment equal amounts of apatite. As expected, the fungi took up the phosphorus-laden compound through their networks but didn’t grow much, choosing instead to store much of the nutrient rather than trade it.
Then came the part inspired by Piketty’s work on economics. Kiers and Whiteside added more phosphorus to one compartment than the other, setting up resource inequalities, with one fungal group controlling up to 90% of the element. The fungi responded by trading with the plants far more than when phosphorus was evenly distributed in the environment.
Most impressively, the fungi moved nutrients from the “rich” to the “poor” region and grew faster in the poor region. Kiers’ team believes that’s because the fungi could extract a higher “price” from the plants in the form of carbon-rich sugars where phosphorus was scarce — though Kiers notes that they couldn’t track the carbon directly.
“This is totally opposite of what we had anticipated,” she said. She thought trading would be highest where nutrients were already abundant.
The demonstration impressed others. “We often think of fungi or other microbes as not particularly intelligent,” said Kabir Peay, a biologist at Stanford University. “This study goes to show that across these networks, one of the reasons they can be so successful is that they can make what seem to be fairly sophisticated decisions about where to allocate resources to optimize the return they get.”
But scientists must take care when applying market concepts to the biological word, noted Ronald Noë, a biologist and professor emeritus at the University of Strasbourg in France who helped pioneer biological market theory in studies of primates, and who assisted Kiers’ team with the economic analysis. “What they describe is a mechanism by which you could be a trader in the market — you can see how they could do it. But they didn’t actually prove that they did it,” Noë said. “If there would be a market, the fungus would bring its nutrients from one plant to the other. But in the experiment, there’s only one plant. The fungus is not choosing.”
The experiment revealed a second surprise. Phosphorus did not just flow from the rich region to the poor one. Kiers and Whiteside caught some of the glowing nutrients oscillating back and forth through the network every five minutes in a regular rhythm.
The scientists don’t know what these oscillations mean. But they do know that oscillations are common ways to encode information. For example, radios work by encoding information in oscillations of radio waves, which are low-frequency electromagnetic waves. Could the fungal oscillations be a form of information transfer across the network?
There is, in fact, strong evidence that information flows across fungal networks. In 2013, David Johnson, a biologist then at the University of Aberdeen in Scotland, discovered that bean plants attacked by aphids sent chemicals underground through fungal networks and into nearby plants that were then alerted to the presence of the pests. Simard has found similar chemical releases in the forests she studies.
But in those studies, scientists inferred what was happening underground from measurements of chemicals in trees. Left unanswered was whether fungal networks are merely conduits of plant-to-plant signals, or if they can process the information they receive. If someone were able to prove the latter, said Erik Verbruggen, a biologist at the University of Antwerp in Belgium who did his doctorate with Kiers, “that would be quite extraordinary.”
Kiers has illuminated the fungal network for the first time. Her next goal is to narrow the gulf between her experimental setups and the complexity of nature. For example, Kiers’ petri dishes flatten the plant-fungus world into two dimensions, but in real soils, fungal networks are 3D. Different species’ networks overlap and interweave, making the wood wide web more like a wood wide tangle with dozens of independent wiring schemes.
“I’d be the first to admit that this is incredibly artificial,” Kiers said of her lab setup. “But that’s actually the beauty of it. We’re starting to deconstruct [the fungal network] piece by piece.”
Kiers is now teaming up with the fluid dynamics researcher Howard Stone of Princeton University, the biophysicist Tom Shimizu of AMOLF and VU Amsterdam, and the network ecologist Hirokazu Toju of Kyoto University. (Their work is funded by a grant from the Human Frontier Science Program to encourage international collaborations on high-risk projects.) They plan to use microfluidic tools to create intricate 3D environments that more closely resemble real-world fungal networks. Stone hopes to augment Kiers’ quantum-dot tags with other techniques for tracking resources such as the carbon that plants trade; some commentators critiqued Kiers’ inequality study for failing to account for that carbon.
“There’s a whole system that we have to set up, including imaging and understanding and possibly modeling, that no one’s done,” Stone said.
For Kiers, the collaboration promises further revelations about how much we’ve misjudged our microbial counterparts.
“Even I, still to this day, underestimate fungi,” she said.
Corrections added Sept. 9, 2019: The caption of the photograph of the segmented petri dish was revised to more accurately describe the experiment shown. The affiliation of Tom Shimizu was also expanded to include his work at AMOLF.