The Epigenetic Secrets Behind Dopamine, Drug Addiction and Depression
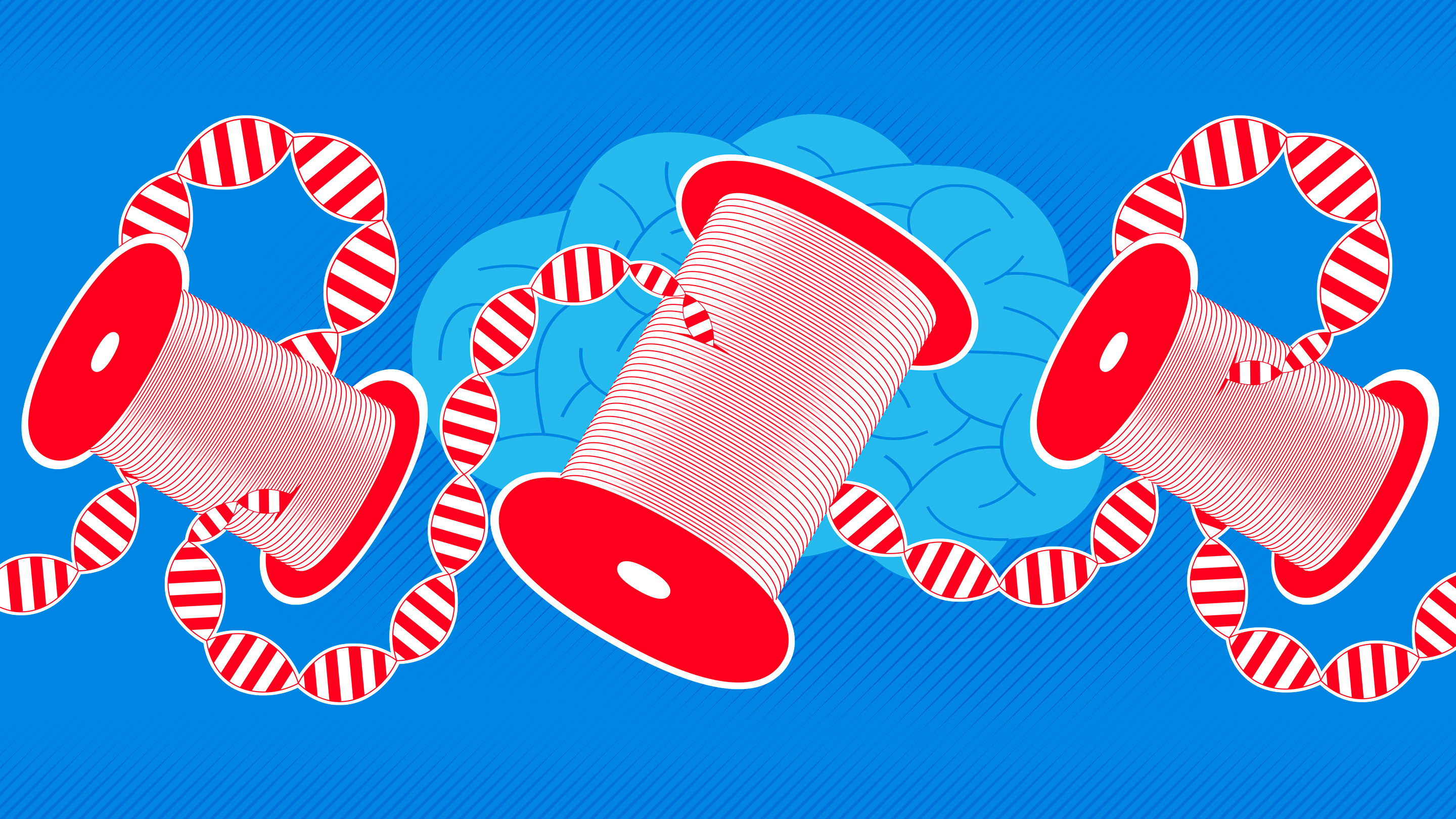
James O’Brien for Quanta Magazine
Introduction
As I opened my copy of Science at home one night, an unfamiliar word in the title of a new study caught my eye: dopaminylation. The term refers to the brain chemical dopamine’s ability, in addition to transmitting signals across synapses, to enter a cell’s nucleus and control specific genes. As I read the paper, I realized that it completely upends our understanding of genetics and drug addiction. The intense craving for addictive drugs like alcohol and cocaine may be caused by dopamine controlling genes that alter the brain circuitry underlying addiction. Intriguingly, the results also suggest an answer to why drugs that treat major depression must typically be taken for weeks before they’re effective. I was shocked by the dramatic discovery, but to really understand it, I first had to unlearn some things.
“Half of what you learned in college is wrong,” my biology professor, David Lange, once said. “Problem is, we don’t know which half.” How right he was. I was taught to scoff at Jean-Baptiste Lamarck and his theory that traits acquired through life experience could be passed on to the next generation. The silly traditional example is the mama giraffe stretching her neck to reach food high in trees, resulting in baby giraffes with extra-long necks. Then biologists discovered we really can inherit traits our parents acquired in life, without any change to the DNA sequence of our genes. It’s all thanks to a process called epigenetics — a form of gene expression that can be inherited but isn’t actually part of the genetic code. This is where it turns out that brain chemicals like dopamine play a role.
All genetic information is encoded in the DNA sequence of our genes, and traits are passed on in the random swapping of genes between egg and sperm that sparks a new life. Genetic information and instructions are coded in a sequence of four different molecules (nucleotides abbreviated A, T, G and C) on the long double-helix strand of DNA. The linear code is quite lengthy (about 6 feet long per human cell), so it’s stored neatly wound around protein bobbins, similar to how magnetic tape is wound around spools in cassette tapes.
Inherited genes are activated or inactivated to build a unique individual from a fertilized egg, but cells also constantly turn specific genes on and off throughout life to make the proteins cells need to function. When a gene is activated, special proteins latch onto DNA, read the sequence of letters there and make a disposable copy of that sequence in the form of messenger RNA. The messenger RNA then shuttles the genetic instructions to the cell’s ribosomes, which decipher the code and make the protein specified by the gene.
But none of that works without access to the DNA. By analogy, if the magnetic tape remains tightly wound, you can’t read the information on the cassette. Epigenetics works by unspooling the tape, or not, to control which genetic instructions are carried out. In epigenetic inheritance, the DNA code is not altered, but access to it is.
This is why cells in our body can be so different even though every cell has identical DNA. If the DNA is not unwound from its various spools — proteins called histones — the cell’s machinery can’t read the hidden code. So the genes that would make red blood corpuscles, for example, are shut off in cells that become neurons.
How do cells know which genes to read? The histone spool that a specific gene’s DNA winds around is marked with a specific chemical tag, like a molecular Post-it note. That marker directs other proteins to “roll the tape” and unwind the relevant DNA from that histone (or not to roll it, depending on the tag).
It’s a fascinating process we’re still learning more about, but we never expected that a seemingly unrelated brain chemical might also play a role. Neurotransmitters are specialized molecules that transmit signals between neurons. This chemical signaling between neurons is what enables us to think, learn, experience different moods and, when neurotransmitter signaling goes awry, suffer cognitive difficulties or mental illness.
Serotonin and dopamine are famous examples. Both are monoamines, a class of neurotransmitters involved in psychological illnesses such as depression, anxiety disorders and addiction. Serotonin helps regulate mood, and drugs known as selective serotonin reuptake inhibitors are widely prescribed and effective for treating chronic depression. We think they work by increasing the level of serotonin in the brain, which boosts communication between neurons in the neural circuits controlling mood, motivation, anxiety and reward. That makes sense, sure, but it is curious that it usually takes a month or more before the drug relieves depression.
Dopamine, on the other hand, is the neurotransmitter at work in the brain’s reward circuits; it produces that “gimme-a-high-five!” spurt of euphoria that erupts when we hit a bingo. Nearly all addictive drugs, like cocaine and alcohol, increase dopamine levels, and the chemically induced dopamine reward leads to further drug cravings. A weakened reward circuitry could be a cause of depression, which would help explain why people with depression may self-medicate by taking illicit drugs that boost dopamine.
But (as I found out after reading that dopaminylation paper), research last year led by Ian Maze, a neuroscientist at the Icahn School of Medicine at Mount Sinai, showed that serotonin has another function: It can act as one of those molecular Post-it notes. Specifically, it can bind to a type of histone known as H3, which controls the genes responsible for transforming human stem cells (the forerunner of all kinds of cells) into serotonin neurons. When serotonin binds to the histone, the DNA unwinds, turning on the genes that dictate the development of a stem cell into a serotonin neuron, while turning off other genes by keeping their DNA tightly wound. (So stem cells that never see serotonin turn into other types of cells, since the genetic program to transform them into neurons is not activated.)
That finding inspired Maze’s team to wonder if dopamine might act in a similar way, regulating the genes involved in drug addiction and withdrawal. In the April Science paper that so surprised me, they showed that the same enzyme that attaches serotonin to H3 can also catalyze the attachment of dopamine to H3 — a process, I learned, called dopaminylation.
Together, these results represent a huge change in our understanding of these chemicals. By binding to the H3 histone, serotonin and dopamine can regulate transcription of DNA into RNA and, as a consequence, the synthesis of specific proteins from them. That turns these well-known characters in neuroscience into double agents, acting obviously as neurotransmitters, but also as clandestine masters of epigenetics.
Maze’s team naturally began exploring this new relationship. First they examined postmortem brain tissue from cocaine users. They found a decrease in the amount of dopaminylation of H3 in the cluster of dopamine neurons in a brain region known to be important in addiction: the ventral tegmental area, or VTA.
That’s just an intriguing correlation, though, so to find out if cocaine use actually affects dopaminylation of H3 in these neurons, the researchers studied rats before and after they self-administered cocaine for 10 days. Just as in the human cocaine users’ brains, dopaminylation of H3 dropped within the neurons in the rats’ VTA. The researchers also found a rebound effect one month after withdrawing the rats from cocaine, with much higher dopaminylation of H3 found in these neurons than in control animals. That increase might be important in controlling which genes get turned on or off, rewiring the brain’s reward circuitry and causing an intense drug craving during withdrawal.
Ultimately, it looks as though dopaminylation — not just typical dopamine functioning in the brain — may control drug-seeking behavior. Long-term cocaine use modifies neural circuits in the brain’s reward pathway, making a steady intake of the drug necessary for the circuits to operate normally. That requires turning specific genes on and off to make the proteins for those changes, and this is an epigenetic mechanism driven by dopamine acting on H3, not a change in DNA sequence.
To test that hypothesis, the researchers genetically modified H3 histones in rats by replacing the amino acid that dopamine attaches to with a different one it doesn’t react with. This stops dopaminylation from occurring. Withdrawal from cocaine is associated with changes in the readout of hundreds of genes involved in rewiring neural circuits and altering synaptic connections, but in the rats whose dopaminylation was prevented, these changes were suppressed. Moreover, neural impulse firing in VTA neurons was reduced, and they released less dopamine, showing that these genetic changes were indeed affecting the brain’s reward circuit operation. This might account for why people with substance use disorder crave drugs that boost dopamine levels in the brain during withdrawal. Finally, in subsequent tests, the genetically modified rats exhibited much less cocaine-seeking behavior.
To put it plainly, the discovery that monoamine neurotransmitters control epigenetic regulation of genes is transformative for basic science and medicine. These experiments show that the tagging of H3 by dopamine does indeed underlie drug-seeking behavior, by regulating the neural circuits operating in addiction.
And, equally exciting, the implications likely go well beyond addiction, given the crucial role of dopamine and serotonin signaling in other neurological and psychological illnesses. Indeed, Maze told me that his team’s latest research (not yet published) has also found this type of epigenetic marking in the brain tissues of people with major depressive disorder. Perhaps this connection even explains why antidepressant drugs take so long to be effective: If the drugs work by activating this epigenetic process, rather than just supplying the brain’s missing serotonin, it can take days or even weeks before these genetic changes become apparent.
Looking ahead, Maze wonders if such epigenetic changes might also occur in response to other addictive drugs, including heroin, alcohol and nicotine. If so, medicines based on this newly discovered epigenetic process could eventually lead to better treatments for many types of addiction and mental illnesses.
In a commentary accompanying the research, Jean-Antoine Girault of Sorbonne University in Paris made a final, intriguing observation. We know that typical neural impulse firing works by causing a ripple effect of dynamic changes in calcium concentration inside neurons that eventually reach the nucleus. But Girault noted that the enzyme that catalyzes the attachment of dopamine to H3 is also regulated by levels of intracellular calcium. In this way, electrical chatter between neurons is relayed to the nucleus, suggesting that neural activity — driven by a behavior — could attach the dopamine epigenetic marker to genes responsible for drug-seeking behavior. That’s how the experiences one has in life can select which genes get read out, and which do not. Lamarck would be proud.
This article was reprinted in Italian at le Scienze and in German at Spektrum.de.