The Quest for Simple Rules to Build a Microbial Community
Introduction
Bacteria don’t throw dinner parties or tell jokes, but they are social in their own way. When the presence of food gives them an opportunity to grow, reproduce and evolve, they will rapidly, even eagerly, form communities. Like a port city springing up along a waterway, a diverse community of bacteria and other microbes will recognize a good situation for growth and build itself up.
Each bacterial city has an origin story. A vat of wine fermenting for months, a biofilm in the lungs of a cystic fibrosis patient and a sulfur-rich hot spring all started with a set of founder cells that proceeded to form a robust network of interacting species. These communities can perform biochemical functions that no single species can do on its own. It takes a quorum of Lactococcus and Streptococcus strains working together to give cheddar cheese its texture and tang. Different combinations of gut microbiota can enhance or blunt the effectiveness of a pill.
However, there are no obvious rules to explain how a bacterial community assembles or why certain species thrive. Most biologists, when faced with describing a community of organisms, catalog the roster of species present. But the number of bacterial species is so vast, their life spans so short and the differences between any two species so minute that species names don’t necessarily provide useful information.
That’s why a group of physicists turned microbiologists are trying to use genome sequencing techniques on a massive scale to uncover any universal rules that might govern bacterial communities — a big-data approach to microbes. Instead of calling out species by name, they are focusing on what the organisms do, with the goal of recognizing what roles are essential within a given community.
“There is redundancy — like, two species can perform the same function — and the same species can perform different functions depending [on] if you change the environment,” said Otto Cordero, a microbiologist at the Massachusetts Institute of Technology. “Taxonomy is not as informative as function.”
Last year in Cordero’s lab, research led by the microbiologist Matti Gralka identified a set of microbial functions that could be predicted without species information. After characterizing the metabolisms of 186 different bacterial strains collected from the Atlantic Ocean, he found that he could predict a given microbe’s basic food preferences based on its genome alone.
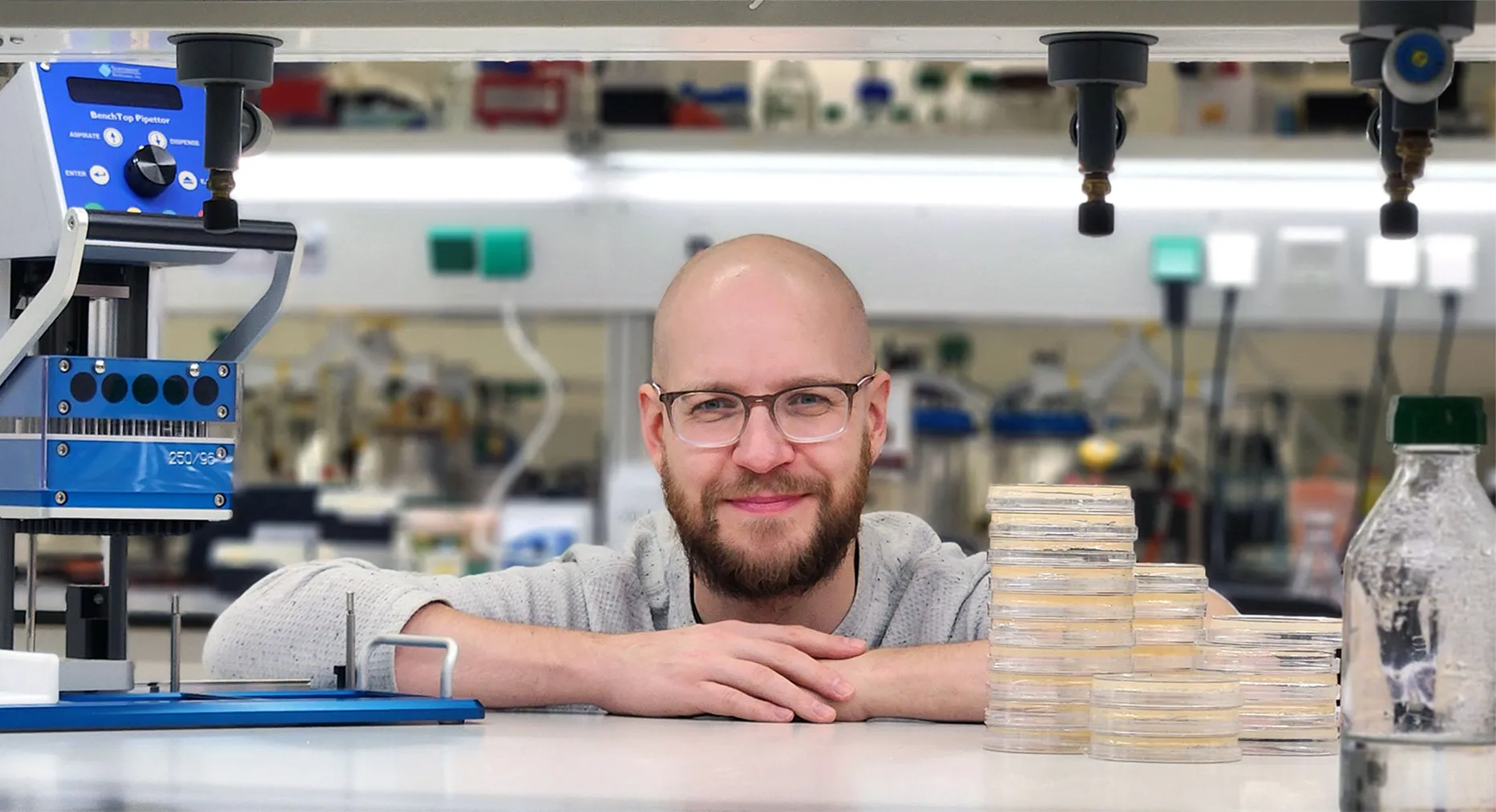
In new research, the microbial ecologist Matti Gralka found that he could predict a microbe’s food preferences based solely on the molecular makeup of its genome.
Dr. Rachel Szabo
This pattern allows researchers to bypass the gene sequences involved in breaking down one food source or another. Gralka’s team discovered that they could predict the preferred food merely by measuring the molecular composition of the genome. The findings were published in Nature Microbiology.
While the field is in its infancy, the microbial ecologists are looking for ways to quickly assess and describe naturally occurring microbial communities, whether in a wild environment or in a hospital. By developing a theory of microbial assembly, they hope they can learn to see the largely invisible and rapidly shifting microscopic ecologies unfolding all around us.
A Field Without a Theory
Microbiology was limited for centuries by the extent of scientists’ ability to see what was in front of them. Even in the early 2000s, if a microbiologist spilled a bacterial community onto a petri dish, it was a monumental task to identify the diverse species, subspecies and strains within it. There were too many organisms mixed together, ebbing and flowing over time as available food sources shifted and species lived and died. Scientists could do little more than identify individual colonies one at a time by shape, color, morphology and nutrient requirements.
Until recent years, this left the field with little defining theory to explain how microbiomes assemble and no sturdy axioms for interpreting experimental results. In 2007, a group of microbiologists wrote in Nature Reviews Microbiology that this absence of theory stemmed from both a lack of data and a fieldwide inability to apply ecological theory to the microscopic world. Without theory, a scientific field has no structure, no form and no predictive power, they argued. A microbial ecologist could make any observation about a community; without theory to explain its importance, anything could be true.
“Sometimes we complain that things are not surprising in microbial ecology,” said Alvaro Sanchez, a microbial ecologist at the Institute of Functional Biology and Genomics, a joint institute of the Spanish National Research Council and the University of Salamanca. “We don’t have strong priors. We don’t have a predictive theory, and so nothing is surprising.”
New genetic tools, however, have led to new ways of describing microbial communities. Sanger sequencing, which for decades was the fastest method for gene sequencing, was only able to identify microbes one by one. Then, in the mid-2000s, high-throughput sequencing technology became available, and in the 2010s it became reasonably affordable. Microbiologists could identify species by whatever DNA was available in the sample.
Microbial ecologists went wild with it. “People were sequencing the hell out of everything,” said Glen D’Souza, a microbial ecologist at the Swiss Federal Institute of Technology Zurich. “The field was dominated by describing who was there — this bug was in this environment; this bug was in that environment.”
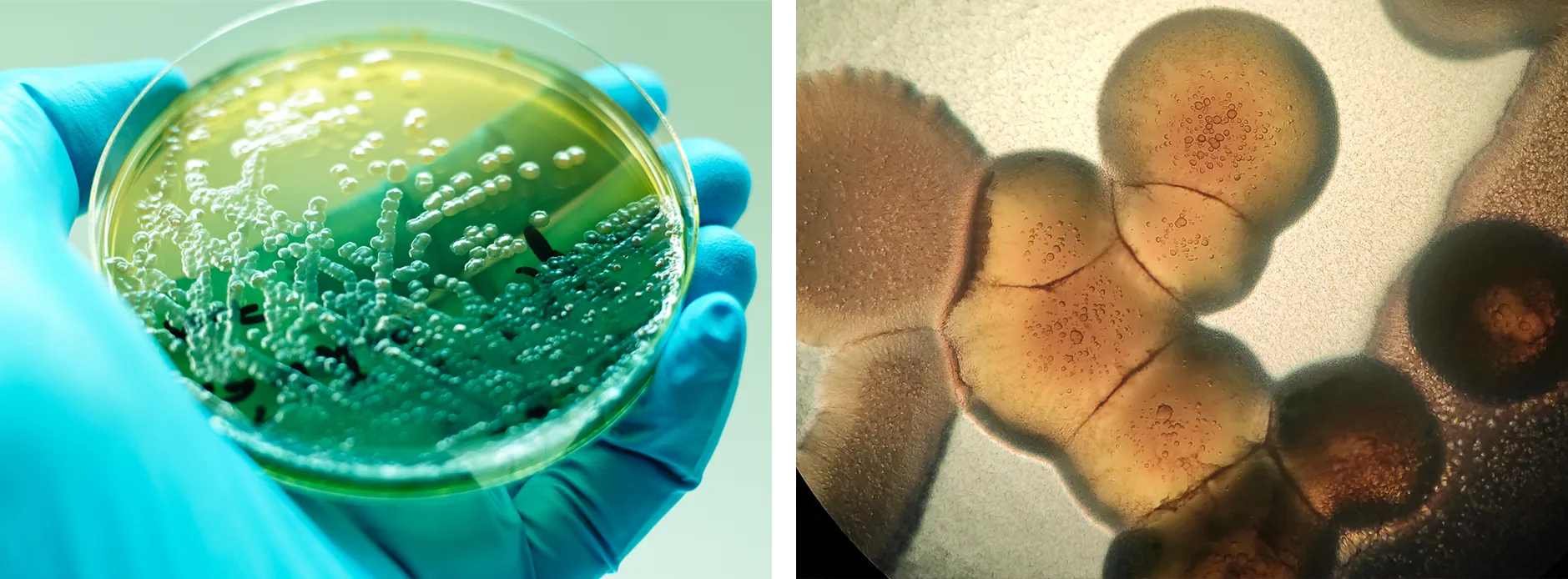
Identifying bacteria grown on a plate (left) or observed under a microscope (right) is a monumental task. New high-throughput genomics tools are giving scientists a much-improved view into microbial communities.
Shutterstock
Suddenly, a glut of data revealed hitherto unknown microbial diversity. In 2009, fewer than 1,000 bacterial genomes had been completely sequenced. By 2014, there were more than 30,000. That figure has since ballooned: At the end of 2023 there were 567,228 complete bacterial genomes, easily browsable and available for cross-reference. Today bacteria account for nearly 80% of all available genomic data.
“People just had no idea how many species there would be,” said Gralka, who now runs his own lab at VU University in Amsterdam. “You can’t tell them apart very well under the microscope.”
However, identifying individual bacterial species in a community can tell scientists only so much. Their names don’t necessarily say much about what each bug is contributing or how the community fits together.
“These communities are high-dimensional,” said Jacopo Grilli, a theoretical microbial ecologist and ex-physicist at the Abdus Salam International Center for Theoretical Physics in Trieste, Italy. “If we try to understand [them], we have to deal with the fact that there are many, many populations, many different species — whatever ‘species’ means — in these communities. All these species have all their own peculiarities, and somehow they’re coexisting.”
In 2018, a Science paper by Sanchez and his team gave microbiologists permission to simplify their thinking. Their breakthrough research showed that if you took a step back and let highly specific details, like exact species names, melt away, you could better understand the logic of a bacterial community, as if you were viewing an abstract painting from a distance.
Like Grilli, Sanchez was a physicist before turning to microbial ecology. “I decided to start working on ecology and microbial communities because I noticed that at the quantitative level, it was an area that had not been as well studied as evolution,” Sanchez said.
For the study, his lab grew wild bacteria cultured from dead leaves and soil around New Haven, Connecticut. They found that given the same set of environmental conditions — the same carbon sources, temperature, acidity and so on — any microbial community will arrive at roughly the same functional composition, no matter how it started. In his experiments, with every population, the same niches appeared and were filled over and over, though not necessarily by the same species of bacteria.
The research changed how microbiologists looked at community. When Sanchez compared communities sampled from the same environment, the names of the bacteria were always different, D’Souza said. “But if you look at the functional gene content, like who does what? That is surprisingly similar,” he said. “So it doesn’t matter who you are; what you do matters.”
The Genome’s Predictive Power
In 2018, Gralka had just arrived in Boston to work as a postdoc in Cordero’s lab at MIT. He’d started out as a biophysicist, studying the physical properties of cells, individually and in aggregates. He had decided to join Cordero’s research program because the two researchers had similar visions: to develop a quantitative, bird’s-eye understanding of microbial communities.
Cordero had a freezer stocked with Atlantic Ocean microbes, which his lab had used to make an interesting discovery about how microbial communities form around food sources, published in Current Biology in 2019. They had dropped balls of chitin — a polymer of repeating sugar molecules that makes up insect shells — into cultures of bacteria grown from the marine samples. When the scientists fished the balls back out, they looked at what communities had formed. Chitin-eating microbes were predictably clinging to the chitin — but there were also bacteria that didn’t eat chitin. Those bacteria seemed to eat the byproducts cast off by the chitin-eaters. The chitin-eaters and byproduct-eaters had formed a community.
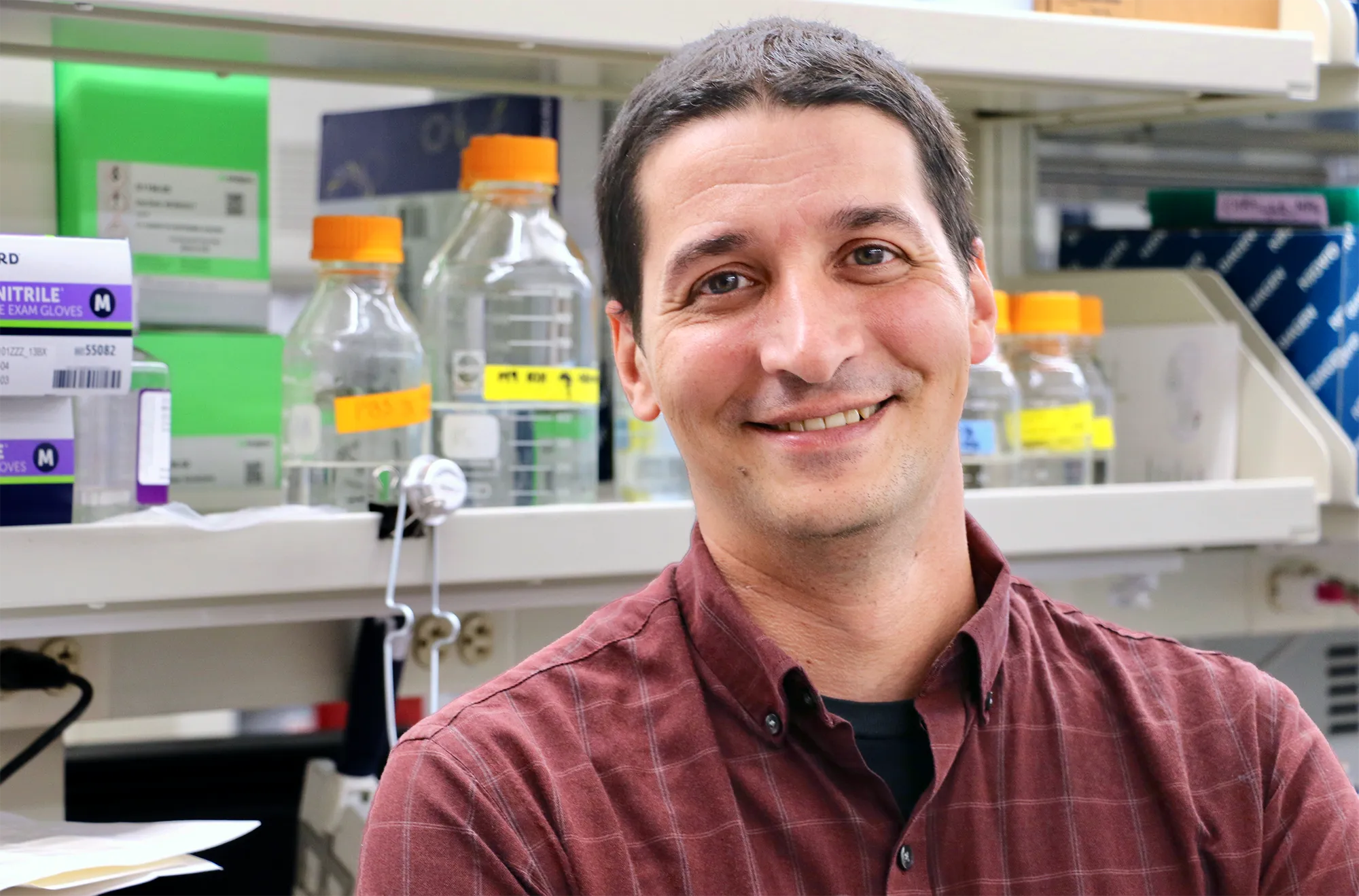
In 2018, the microbial ecologist Alvaro Sanchez found that the niches within a microbial community are predictable, given the same starting conditions.
Jon Atherton
This intrigued Gralka. It seemed possible that the type of community could be predicted from its food sources alone: from the original food source, and then from the new sources created when the initial bacteria broke it down. He wondered whether he could predict the arc of a microbial community’s changes if he controlled its starting conditions.
Then, right when he joined Cordero’s lab, “a paper came out of Alvaro [Sanchez]’s lab that made a pretty big splash,” Gralka said — the 2018 work showing that predictable microbial niches appear that can be filled by many different species. The idea that function mattered more than species made sense to him. “In soil you sometimes find thousands of different bacteria. Then that very quickly opens up questions,” he said. “How are there thousands of species? Surely there aren’t thousands of different niches.”
Combining those two insights from Cordero and Sanchez, Gralka wondered if he could not only predict a microbial community from its starting food source, but also infer niches from the bacteria’s genomes.
Gralka sampled Cordero’s freezer. First, he needed to characterize the bacteria based on what foods they preferred. Using high-throughput tools, he grew 186 different bacterial species in cultures supplemented with 135 different food sources. All told, Gralka measured the growth rates of more than 25,000 bacterial samples.
There’s as much variety in 186 bacterial species as there is in 186 different humans, and like humans, bacteria each have their own patterns and habits. Some of Gralka’s bacteria grew rapidly on sugars, and others grew rapidly on acids, including organic acids like citric acid as well as amino acids, the building blocks of proteins. Using that data, Gralka placed the species on what he called a sugar-acid axis based on their preferences.
Then he sequenced the DNA of all 186 species to see how they were related evolutionarily. Gralka was surprised to see that closely related species within the same phylogenetic families often had different metabolic preferences. For example, the order of rod-shaped bacteria Alteromonadales contained within it the acid-eaters Colwellia, the sugar-eaters Paraglaciecola and the less picky Pseudoalteromonas, which ate both. That supported the broader idea that species names don’t convey much information about the bacteria’s function within a given microbial community.
Then Gralka’s analysis dug deeper into the bugs’ DNA. To relate the genome to metabolic function, he sought out genes known to be involved in digesting and metabolizing sugars, and did the same for acids. He found that the number of sugar- or acid-eating genes predicted where each microbe fell on the sugar-acid spectrum: The more genes a species had for one process or the other the more likely it was to land on that end of the axis. The findings suggested that microbiologists could roughly establish the metabolism of a community by searching for sequences of certain genes.
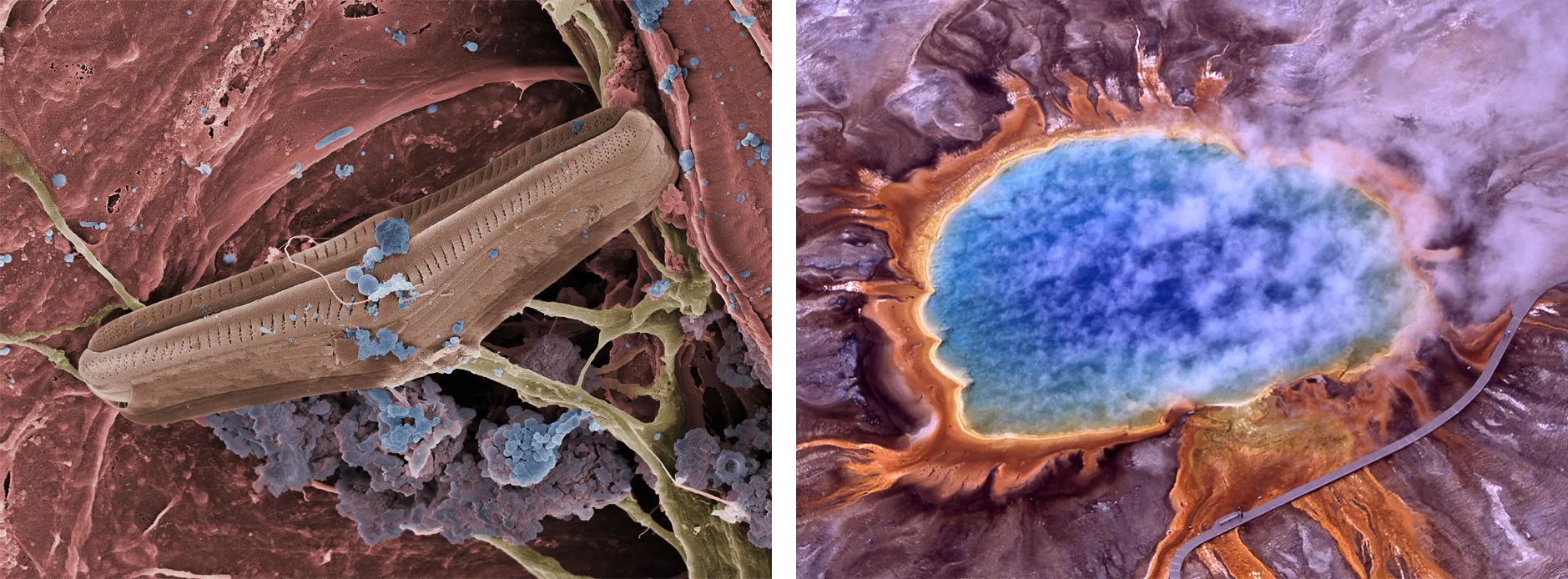
Microbial communities are key players in every ecological cycle on Earth and can be found just about everywhere — even in extreme habitats like the sulfur-rich hot springs of Yellowstone National Park.
Ted Kinsman/Science Source; National Park Service
Then he found something more surprising. Ignoring the actual gene sequences, he looked directly at the molecular breakdown of a strain’s DNA. In the double helix of DNA, the four types of bases in the opposing strands are paired, with guanine (G) bound to cytosine (C) and thymine (T) bound to adenine (A). Unexpectedly, the genomes of acid-eaters had an average of 55% GC content, while sugar-eaters’ GC content averaged around 40%. To confirm that this correlation wasn’t a quirk of his particular microbial community, Gralka analyzed a larger data set of thousands of reference genomes from across the bacterial tree of life. The pattern held up: Acid specialists generally had a higher GC content than sugar specialists.
This rule seemed unimaginably simple. The chemistry of a bacterium’s DNA predicted its niche in the community. Gralka could identify whether a species primarily ate sugars or acids based on the content of its genome alone, without examining its genes at all. Statistics and genomics found simple order where taxonomy didn’t see any.
Predicting the Microbial Future
The work lays the foundation for a new science of making practical predictions about microbial communities. Say a pipeline leaks and spills crude oil in a forest; a microbiologist or environmental scientist might want to know what bacteria will pop up to eat that oil. A doctor might want to know how the gut microbiome of a patient could change over the course of a disease, and potentially use that prediction to prescribe specific antibiotics or other medicines.
Many questions can be answered and problems solved if researchers can quickly estimate the functions of a microbial community. “In my lab, we call it the coach’s dilemma,” Sanchez said. “You have a bunch of players, and you want to figure out who you should put on the court if you want to maximize your score. I have this list of 100 strains; I want to put them in a bioreactor, and I want to make as much ethanol as possible. So which strains should I put in?”
The rules that microbial ecologists are uncovering can’t answer that question yet. However, a quick assessment of microbial metabolism — or a working theory of bacterial communities and their genes — could someday be used to study and manage a world of ecological processes, Gralka said.
Microbial communities are key players in every ecological cycle on Earth. When a tree falls in a forest, a litany of fungi and bacteria assemble to eat and decompose it, returning the tree’s components to global nutrient cycles. With the concepts introduced by Gralka, Sanchez, Cordero and other microbial ecologists, this new community’s niches are predictable. Wood is mostly composed of cellulose and hemicellulose, which are glucose polymers; therefore, a functioning community ripe for participating in woodland decomposition would host sugar-eating bacteria, be abundant in sugar-digesting genes, and have genomes composed of a lower proportion of GC molecules. A sudden and mysterious spike in acid-eaters could be a sign of something amiss, Gralka suggested.
The sugar-acid axis is only one kind of community niche that these microbial ecologists want to identify. Cordero offered the forest ecosystem as an example of their ultimate aim. Ecologists have defined many general traits and functions that are shared between forests and differ among them, enabling comparison and prediction.
“How much biomass is on the leaves versus the trunk? [It] turns out that plants that have huge leaves do more respiration in tropical environments,” Cordero said. “How deep are the roots? That tells you how much nutrients they can take from the environment. How fast will they grow? How tall are they? How good are they [at] competing for light?” Knowing even a few of those variables can tell us a lot about a forest’s dynamics.
Cordero doesn’t know what the analogous traits might be for microorganisms and their communities. Many bacterial niches are surely related to their metabolism and byproducts, but there are other angles to consider. “If we had ways to learn what these variables are … and ways to identify them systematically, that would be amazing,” he said.
In a sense, these scientists are ecologically mapping microbial communities for the very first time. Their work proposes a new view into what a microbial community actually is — showing that what microbes are is best defined as what they do.
Editor’s note: Cordero leads the Simons Collaboration on Principles of Microbial Ecosystems, a research program supported by the Simons Foundation, which also funds this editorially independent magazine. Simons Foundation funding decisions have no influence on our coverage.